All published articles of this journal are available on ScienceDirect.
The Human Immune System toward Staphylococcus aureus
Abstract
The immune system is responsible for protecting the host from pathogens, and it has evolved to deal with these pathogens. On the other hand, the co-evolution of pathogenic bacteria with hosts has led to the rise of an array of virulence genes that enable pathogen bacteria to evade or modulate the immune system. Staphylococcus aureus is a significant pathogen of humans that encodes several virulence factors that can modulate or evade from the innate and adaptive arm of the immune system. Overall, the immune reaction toward S. aureus contributes to stimulate innate and adaptive reactions. A profound understanding of the immune response to S. aureus infections will be critical for the development of vaccines and novel therapies. In this review, we summarized and discussed the novel information about the human immune system against S. aureus.
1. INTRODUCTION
Staphylococcus aureus, a Gram-positive anaerobic bacterium, is the most important medically species in the genus of Staphylococcus [1]. This organism develops a wide variety of infections ranging from simple skin infections (like corks, scabies, boils, and abscess) to life-threatening diseases (like endocarditis, pneumonia, osteomyelitis, meningitis, septicemia, toxic shock syndrome) [2]. Methicillin-resistant S. aureus (MRSA) that is resistant to most antibiotics is considered a threat to public health [3]. Despite their obvious pathogenic potential, S. aureus strains have the potential to co-exist with their host as a commensal, with approximately 30% of the human population colonized at the mucocutaneous section [4, 5]. The human immune system detects pathogens and destroys them [6, 7]. In this regard, the immune system includes innate and adaptive sections [6, 7]. The innate or nonspecific immune system or natural immunity is the first level of protection and consists of cells that commonly find and destroy pathogens in the body [8]. As the primary class of defense in bacterial infections, the innate immune reactions are rapidly induced by pattern recognition routes that recognize non-specific markers of bacterial infection [9]. A major consequence is the stimulation of phagocytic immune cells like neutrophils and macrophages [9]. The innate immune cells can be induced via Toll-like Receptors that enhance antibacterial reactions, stimulate inflammation, and trigger effector cells. This process finally results in the production of interferons, pro-inflammatory as well as cytokines. Pro-inflammatory cytokines are produced by resident host cells that cause the fever [10].
Acquired (adaptive) host immunity is the second type of immunity that is much more evolved than innate immunity and is induced with each encounter with infectious agents and with each repeated exposure to a particular pathogen that increases its defense capacity and capabilities [11]. An important feature of this type of immunity is that it has a specific response for each external factor and that it is also a faster and stronger response [11]. Via activation of T cells and antibodies formation by B cells, the adaptive host immune reaction targets particular microbial antigens and could be recalled in subsequent bacterial infections to offer memory toward that specific pathogen [11]. In this review, we summarized and discussed the novel information about the human immune system against S. aureus.
2. INNATE IMMUNE RESPONSES TOWARD S. AUREUS
The skin is the largest organ in the body and the main physical barrier between the organism and its environment [12]. It also plays an active role in the defense of the host and, therefore, plays a role in the development and maintenance of local immune and inflammatory responses [12]. Many immune responses originate from this tissue as the gateway to many alien antigens [12]. The skin contains important cells like melanocytes, keratinocytes, and Langerhans and T lymphocytes. Langerhans is immature Dendritic Cells (DCs) of the immune system and its function is to trap the antigens that enter the host skin [13]. The epidermis layer of skin is organized by keratinocyte cells in various maturation levels, T lymphocytes as well as Langerhans cells. The dermis layer comprises extracellular matrix ingredients, like connective tissues, elastin fibers, and collagen [14]. The fibers offer a building skeleton to human blood vessels, fibroblasts, adipocytes, macrophage cells, DCs, mast cells, T cells, B cells, and plasma as well as Natural Killer (NK) cells [14]. As such, inhabitant human immune cells are plentiful in the skin, and these cells are all contributed to the control of infection of S. aureus strains.
Keratinocyte cells are the central cells of the epidermis and have a protective function against external factors such as bacteria [15]. Keratinocyte cells are usually the first cells that encounter microbial pathogens, and detect Pathogen-Associated Molecular Patterns (PAMPs) through various Pattern-Recognition Receptors (PRRs), like nucleotide-binding oligomerization domain-1 (NOD-1), nucleotide-binding oligomerization domain -2 (NOD -2), TLRs as well as the scavenger receptors CD36 [15-17]. Signaling via these human receptors stimulates transcription factors like AP1, NF-kB as well as CREB to produce chemokines, cytokines, and antimicrobial effectors, like Antimicrobial Peptides (AMPs) and inducible Nitric Oxide synthase [16, 18, 19]. TLR-1, TLR-2, and TLR-6 detect the cell wall components S. aureus, particularly peptidoglycan and lipopeptides [20, 21]. These TLRs apply the signaling adapter MyD88 to stimulate efficient and robust transcriptional programs that trigger inflammatory reactions [22]. TLR-1, TLR-2, and TLR-6, contribute in different stages of infection caused by S. aureus. Primarily, TLR-2 on Keratinocyte cell detect bacterial pathogens to release neutrophil antimicrobial peptides and chemo-attractants, like the defensins and cathelicidin LL-37, which produce pores in the membrane of bacteria [19]. TLR-2 molecules are formed on inhabitant recruited neutrophils, monocytes, and macrophages, which immediately react to S. aureus and further activate cytokine formation and phagocytosis [23]. It is supposed that TLR2 molecules are essential for localized and systemic S. aureus infection. Mouse deficient in TLR-1, TLR-2, TLR-6, and MyD88 is very susceptible to intranasal infection of S. aureus, as documented by the raised load of bacteria, poor inflammatory reactions, and increased morbidity and mortality in different models of disease [24]. In human skin, the TLR2 activity is controversial due to variation in virulence of microbial strains, measured endpoints as well as infectious dose (Fig. 1).
Neutrophils are a type of phagocytic white cells that have chemotaxis and are in the second line of nonspecific defense in the human immune system [25]. The neutrophil is a crucial section of the acute reaction and centrally relevant toward S. aureus [26]. The NOD-1, NOD-2 and intracellular PRRs that recognized microbial peptidoglycan stimulate antimicrobial peptide formation, inflammation, and phagocytic effector activities [27]. NOD-2 molecules detect muramyl-dipeptide from the peptidoglycan of S. aureus [28, 29]. The NOD2-deficient mouse is very susceptible to systemic and skin infections of S. aureus when compared with wild type counterparts [29, 30]. Eventually, scavenger receptors SRBII, CD36, as well as MARCO are required for optimal skin host defense against S. aureus [31, 32]. As a result, CD36 2/2 mouse shows raised bacterial loads and enhances severe dermonecrosis induced by α-toxin [31].
Macrophages have spread throughout the body [33]. Immature macrophages in the bloodstream are called monocytes [33]. Skin-inhabitant macrophage cells assist in the first S. aureus clearance, and in conjunction with perivascular macrophage cells. Moreover, they control the recruitment of monocytes and neutrophils to the location of infection [34]. Dermal macrophage cells could phagocytose and kill S. aureus efficiently by forming antimicrobial peptides, reactive nitrogen, and oxygen species as well as chelating proteins that starve microbes of essential micronutrients [35, 36]. Additionally, dermal macrophage cells release various chemo-attractant molecules that offer signals for the recruitment of neutrophil cells in a manner mediated to MyD88, IL-1-R [37]. Moreover, these cells contribute to the dead cell-clearance at the site of bacterial infection, which is vital for the resolution of the disorder [35, 36]. When the neutrophil cell reaches the location of the infection, it engulfs the S. aureus and attempts to control bacterial growth by forming various antimicrobial effector molecules [35]. The neutrophil is a short-lived immune cell that quickly triggers apoptosis and requires to be eradicated from the location of the infection. Nevertheless, S. aureus cells form different toxins, like Panton-Valentine leukocidin, phenol soluble modulins, α-toxin as well as γ-hemolysin, and that could hasten neutrophil death by stimulating necrosis and cause to liberate the danger-mediated molecular patterns [35, 38]. Danger-mediated molecular patterns freed in S. aureus infection like IL-1α, IL-33, HGMB1, ATP, as well as calprotectin [39]. DCs and Langerhans cells capture the microbial antigens before contact with skin-draining lymph nodes [40, 41]. The study has found that in skin S. aureus infection, Langerhans cells that engulf the bacteria are stimulated by PAMPs, and subsequently migrate to draining lymph nodes where Langerhans cells elicit adaptive reactions specific to S. aureus [42, 43]. Although there are various distinct sub-kinds of dendritic cells in the human skin, its activity against S. aureus infection in the skin is not well-defined [44].
S. aureus cells can be engulfed by receptors that detect non-opsonized and opsonized bacteria [35, 38, 45]. S. aureus cells trigger different antimicrobial effector activities, when coated with opsonins like C3b and IgG [44]. Reactive oxygen species are produced via the actions of myeloperoxidase and NADPH oxidase following phagocytosis, which can directly kill microbes [46]. Nitric oxide molecules are a main reactive nitrogen species that formed from nitric oxide synthase with immunomodulatory and antimicrobial activity [46]. Pharmacologic inhibition and genetic deletion of nitric oxide production render mice susceptible to S. aureus infection [47].
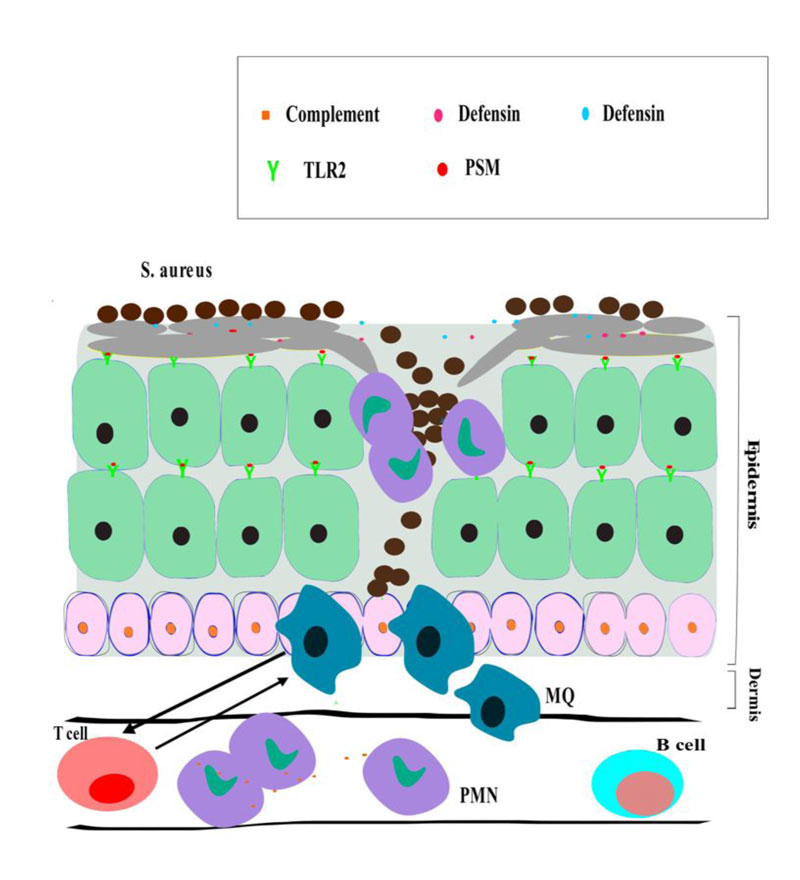
Nevertheless, high levels of nitric oxide could exert anti-inflammatory impacts. High concentrations of nitric oxide can, therefore, predispose to bacterial infection via inhibiting the proliferation of the cell, stimulating host cell death, and preventing TNF-α formation induced by phagocyte and presentation of Ags.
Additionally, S. aureus cells use nitric oxide to proliferate and preclude stimulation of the stress regulon through the fermentation of lactic acid [48, 49].
Neutrophils kill pathogens by degranulation of toxic components [50]. Degranulation stimulates the formation of particular granules containing antimicrobial peptides, like LL37, defensins, and cathelicidin-related antimicrobial peptide. Moreover, degranulation diffuses cathepsin, azurocidin, lysozymes, lactoferrin, elastase, and proteinase-3 [51, 52]. Neutrophils liberate structures of DNA named NET (neutrophil extracellular trap), as an additional effector mechanism to control S. aureus infection, that formed in a mechanism dependent on a TLR2 and MyD88 and is necessary for containing S. aureus in the host skin to prevent bacteremia [53]. NET finites the spread of bacteria because it is rich in antimicrobial agents like cathepsin, antimicrobial peptides, histones, elastase, as well as proteases [54]. Nevertheless, S. aureus cells can destroy NET, and the degradation product 2’-deoxyadenosine that stimulates apoptosis in macrophage cells, which raise bacterial survival in the abscess [55].
Some AMPs exist in the human skin with activity toward S. aureus cells, for example, Alpha-defensins (named HNPs (human neutrophil peptides)), dermcidin, Beta-defensins, cathelicidin, as well as RNase7 [56, 57]. Neutrophil releases high rates of HNPs, which included nearly half of the peptides inside granules of neutrophils [58]. Amongst HNPs, HNP2 peptides have the highest degree of antibacterial role toward S. aureus cells [59]. In humans, there are four human β-defensins (hBDs), including hBD1, hBD2, hBD3, hBD4, which are formed by keratinocytes, macrophages, as well as DCs [60, 61]. Amongst hBDs, hBD3 has a potent activity toward S. aureus [62]. Cathelicidin is also called LL-37 as it has 37 amino acid released from the cationic antimicrobial protein 18 kDa [63] similar to hBD3, which has strong activity against S. aureus [64]. This AMP is formed in neutrophil cells and could be stimulated in keratinocyte cells [64]. Another AMP, RNase 7, is formed by human keratinocyte cells and has activity toward S. aureus [65]. A current work discovered that high levels of RNase7 could stop S. aureus skin colonization in the host stratum corneum [66]. Additionally, dermcidin is formed by eccrine sweat glands with activity toward S. aureus [67]. The keratinocyte cells form the LL-37, hBD2 as well as hBD3 in S. aureus infections for prompt clearance of the bacterial cells [68]. In addition, stimulation of the EGFR (epidermal growth factor receptor) by wounding of skin cause in enhanced hBD3 formation offers another strategy for increasing antibacterial role toward S. aureus [69].
Four PGRPs (peptidoglycan recognition proteins) exist in humans, including PGRP1-4 that secrete proteins [70]. PGRP1 molecules are formed in neutrophil cells, where they bind peptidoglycan S. aureus inside tertiary granules and apply their bactericidal activity [71]. PGRP2 molecules are formed by keratinocyte cells and are distinct that they have the active amidases that cleave the peptidoglycan of S. aureus [72, 73]. Finally, PGRP3 and PGRP4 produce in the hair follicles, epidermis, sebaceous glands, as well as sweat glands and their relevance to S. aureus infection is unknown [74]. Complement activation, as a crucial section of the innate immune defense, triggers cytolytic reactions and acute inflammatory responses. Furthermore, it involved in the regulation of host adaptive immune defenses [75, 76]. The complement is stimulated through particular recognition routes, including the classical and the alternative and the lectin pathway [75]. All pathways form the active protease complexes, the C3 convertase, on the bacterial surface [77]. C3 convertase activity leads the labeling of microbial antigens with a high amount of C3b, as well as iC3b [75]. This process is named opsonization that is crucial for impressive phagocytic uptake through complement receptors by phagocytic cells [78]. It must be noted that a highly particular factor called staphylococcal complement inhibitor produced by S. aureus cells attaches to and stops the role of the C3 convertases on the staphylococcus surface [79].
3. ADAPTIVE IMMUNITY AGAINST S. AUREUS
It is unclear whether the limited association of B cells and S. aureus affects the pathogenesis and/or host immune response, but, it has been shown that the binding of CR2 expressed by B lymphocyte with C3d on the antigen surface has several roles useful for the antibody action [80]. For cognitive B cells, the simultaneous binding of CR2 with C3b with the B lymphocyte antigen receptor complex reduces the B cell activation threshold by several orders of magnitude. It is required for proper B cell activation [80]. On the other hand, non-cognitive B cells in the lymph nodes and spleen of mice play an important role in the transfer of immune complexes containing Immunoglobulin M to follicle DCs [80]. This process is dependent on the receptor and complement that is essential for the efficient formation of the germination center and maturation of the antibody affinity [80]. Unrecognized CR2 B cells attached to antigen-containing immune complexes can also deliver these immune complexes to antigen-specific T cells to enable appropriate T-cell activation [80]. Thus, molecules secreted by S. aureus that inhibit the association of C3d-containing immune complexes with CR2 can influence the host's adaptive immune response against this bacterium [80].
B lymphocytes are immune cells that produce antibodies against pathogens, such as viruses [81]. These lymphocytes are involved in humoral immunity, which, together with cellular immunity, form a specific defense mechanism [81]. B lymphocytes act in a specific way; that is, they identify and eliminate a particular type of alien agent [81]. These cells have a major role in humoral immunity [82]. The primary function of antibody-deficient lymphocytes against antigens is to function as Antigen-Presenting Cells (APCs), and eventually to evolve into memory cells after activation by antigenic interactions [82]. Memory cells produce more antibodies in a shorter time after re-encountering the same antigen [83]. The main activity of B lymphocytes is to form immunoglobulins that neutralize the action of target proteins (like toxin) or opsonize microbial pathogens to optimize clearance and phagocytosis [83]. The significance of antibody-mediated protection toward microbial infectious agents is found by demonstrating this event that the absence of B lymphocyte maturation causes susceptibility against infections with encapsulated bacteria and viruses [84]. The notable absence of raised susceptibility in this regard to S. aureus argues that antibody is un-significant in protection toward infection of S. aureus. Nevertheless, it has found that early cutaneous S. aureus infection could stimulate antibody-mediated support toward a subsequent microbial infection in certain mouse strains, and many pre-clinical works have found at least partial support from subsequent microbial infection subsequent stimulation of antibodies by vaccination [85].
In the opsonophagocytosis, T lymphocyte has a dual function: (1) it is important for the production of opposing antibodies because T cell is essential for maturation of antibody affinity as well as class switches, (2) T cells promote phagocytosis by absorbing neutrophils and macrophages from the bone marrow to the site of infection [86, 87]. As long as the S. aureus resides in the macrophage phagosome, its elimination by T-cell cytokines is more pronounced by IFN-γ [87]. The infected cell must now be lysed by Cytotoxic Cells (CT) cells and/or NK cells [88]. Finally, T cells are essential for the (re) establishment of immune homeostasis by blocking inflammatory processes [88]. Treg regulatory (T-reg) cells specialize in this critical immune function [88]. T lymphocytes proliferate upon binding to the antigen, producing a variety of T cells, including some lethal T and some T memory [89, 90]. Lethal T cells directly invade virus-infected cells and cancer cells and kill them [90]. That is why this type of immune response to cellular immunity is known [90]. T lymphocyte is thymic-derived cells that form particular TCR receptors that detect peptides derived from antigen in terms of MHC molecules on antigen APC cells. Similar to antibody and B lymphocytes, a case could be formed for an activity for T lymphocytes in S. aureus infection according to the presence of detectable T lymphocytes reactions in humans and the capacity of the bacteria to modulate T lymphocytes as exemplified by their formation of a multitude of T lymphocyte super-antigens [91, 92]. Nevertheless, it has been found that T lymphocyte is not essential for protection toward S. aureus in mice [93]. Different sets of T lymphocyte have differing activities, and further nuanced activities for these sets have become a document in mouse evaluations and with the detected susceptibility to various staphylococcal infections of individuals with Human Immunodeficiency Viruses (HIV) disorder [94]. Most of T lymphocytes like CD4 (positive) and CD8 (positive) T lymphocytes have long been detected as a main cellular weapon of adaptive immunity of host [95]. The main activity of CD8 (positive) T lymphocytes is to kill intracellular microbial pathogens by the cytolytic killing of the infected human cell [96]. Consistent with S. aureus cells being an early extracellular microbial pathogen, the clear activities for CD8 (positive) T lymphocytes have not been found, although activation of CD8 positive T lymphocytes could be found in staphylococcal super-antigen exposure and S. aureus infection. Naive CD4 positive T lymphocytes are polarized against different effector activities mediated to the cytokine milieu in which stimulation of their TCR receptor occurs. These Th lymphocytes are functionally determined by their cytokine formation profiles. A rate of these polarized lymphocytes will exist in the human as memory lymphocytes awaiting re-stimulation by next exposure to antigen. More recently, in addition to CD4 positive and CD8 positive T lymphocytes, a set of T lymphocytes has been described, such as γδ T lymphocytes, NK cells, and innate lymphoid cells, that involve mostly in the innate immune reactions at mucosal locations instead of memory specific to the antigen, although current works have proposed the potential for γδ T lymphocytes to involve in a memory reaction under some conditions [97].
CONCLUSION
In summary, host immunity in S. aureus infections is tissue-specific and it significantly is mediated to PRRs, like NOD-2, TLR-2, and for particular cytokine signaling mechanisms, like IL-1. Nevertheless, adaptive host immunity (B and T lymphocyte reactions) can impact this defense. Overall, the immune reaction toward S. aureus contributes to the stimulation of both innate and adaptive reactions. Adaptive immunity of the host may impact susceptibility to infections of S. aureus and its ability has specific significance in determining the results of chronic persistent human infections. Finally, a profound understanding of the human immune response to S. aureus infections will be critical for the development of vaccines and novel therapies.
AUTHOR'S CONTRIBUTIONS
R.M, S.K, R.G, S.G prepared the manuscript, and R. R edited the manuscript and H.H designed the figure.
CONSENT FOR PUBLICATION
Not applicable.
FUNDING
None declared.
CONFLICT OF INTEREST
The authors declare no conflict of interest, financial or otherwise.
ACKNOWLEDGEMENTS
Thanks to guidance and advice from the “Clinical Research Development Unit of Baqiyatallah Hospital”. The authors also thank the Department of Microbiology of Hamadan, Hamadan University of Medical Sciences, Iran.