All published articles of this journal are available on ScienceDirect.
Optimization of Phosphate Solubilization Activity of Enterobacter Cloacae and Enterobacter Hormaechei from Rhizosphere
Abstract
Introduction:
The availability of phosphorous to plants for uptake and utilization is limited in soil due to fixation in the form of Fe-P, Al-P, and Ca-P; hence, there is a need for phosphate solubilizing organisms to help improve the availability of phosphorus for plants and enhance their productivity.
Background:
The aim of this study was to assess the growth-promoting potential of phosphate-solubilizing bacteria isolated from leguminous and non-leguminous rhizosphere. A total of 52 bacterial strains were isolated from rhizospheres of leguminous (cowpea, groundnut, and soybean) and non-leguminous (rice, maize, and cassava) plants, using the standard pour plating procedure.
Methods:
The bacterial strains were subjected to preliminary screening for phosphate solubilization potential in solid media, using the degree of solubilization index as an indicator. Further screening of isolates that showed remarkable solubilization index during preliminary screening was carried out in a liquid medium. Optimum conditions (external carbon and nitrogen sources, temperature, pH, and initial inoculum concentration) for phosphate solubilization by the isolates were determined. The isolates were identified using the 16S rRNA polymerase chain reaction. Sequences were deposited in the National Centre for Biotechnology Information database and ascension numbers were obtained.
Results:
The results revealed five bacterial species (two species of Enterobacter hormaechei and two species of Enterobacter cloacae) to show significant phosphate solubilization in liquid and solid media. Although the study revealed no significant difference in phosphate solubilization in the presence of the isolates at the respective carbon and nitrogen sources, the highest solubilization was observed in the medium that contained sodium acetate and ammonium sulphate, respectively. In the presence of carbon or nitrogen sources, no particular trend of increase or decrease in phosphate concentration throughout the incubation period was observed. However, the highest phosphate solubilization was observed at 72 h of incubation. This observation was irrespective of the test isolates. Incubation temperature of 35oC and medium pH of 9 were observed to show significantly higher phosphate solubilization in the presence of the isolates.
Conclusion:
In addition, the study revealed no observed consistent pattern of increase or decrease with the period of incubation at different initial inoculum concentrations. The application of the test isolates as soil inoculants could enhance growth promotion and help improve the yield.
1. INTRODUCTION
Phosphorus is an essential element in that it is required by all plants to complete their life cycle. It is one of the most common metallic elements in the crust of the earth and is present in soils in both inorganic and biological forms [1]. It is used or absorbed by plants in orthophosphate form. In legumes, phosphorus is essential for photosynthesis, the metabolism of carbon, the development of membranes, the transfer of energy, the transmission of signals, and the fixation of nitrogen. It is essential for root multiplication and elongation as well. Phosphorus deficiency has an impact on plant root architecture [2, 3].
In addition, phosphorus is known to enhance crop quality and resistance to plant diseases. Since phosphorus is a structural element of several coenzymes, phospho-proteins, and phospholipids, it also contributes to the genetic information of all living things and participates in the exchange and storage of energy needed for growth and reproduction [4]. Acidic soil is considered very phosphorus deficient in tropical and subtropical regimes with strong phosphorus sorption (fixation) capacity. The majority of the mineral nutrients found in soil solutions are typically present at millimolar concentrations; however, phosphorus is only found in micromolar or smaller concentrations. The strong reactivity of soluble phosphate with other elements is the cause of the low amounts of phosphorus [5].
Several heterotrophic bacteria excrete organic acids that solubilize phosphate and chelated cationic partners of phosphate ions before releasing the phosphate into the solution [6]. Soluble phosphorus is assimilated by microbial inoculants, which also stop it from becoming adsorbent or fixed. These microorganisms have an impact on soil fertility through a variety of mechanisms, including nutrient cycling, mineralization, and decomposition. By converting phosphorus from its organic form to one that is readily available to plants, they contribute to improving phosphorus availability [2, 7].
Despite the fact that the application of chemical fertilizers to soil has the benefit of increasing the yield of crops, the fact that they are not fully degraded in the soil may lead to the problem of soil toxicity and possibly bioaccumulation along the food chain. The application of chemical fertilizers may also be expensive; hence, there is a need for a natural and cheaper method. There is, thus, a need for eco-friendly, cheaper, viable, and degradable alternative methods for phosphorus availability to plants. One such option is the use of phosphate-solubilizing microbes [8].
A variety of soil microorganisms are known to play varied roles that affect phosphorus transformation in soil, and thus influence the availability of phosphorus to plant roots. Effective fixed phosphate solubilizers include fungi, bacteria, and actinomycetes [9]. It is indicated that phosphorus in fertilizer and soil-bound phosphorus can be easily and sustainably dissolved in the presence of phosphate-solubilizing bacteria [10].
Evidence of naturally occurring phosphate solubilizing microbes indicates that bacteria are more efficient than fungi, with Pseudomonas and Bacilli and endosymbiotic rhizobia described as effective phosphate solubilizers. Moreover, a large array of rhizosphere bacteria and fungi, including species of Penicillium, Azotobacter chroococcum, Enterobacter aerogenes, Bacillus subtilis, Bacillus cereus, Bacillus megaterium, Microbacterium laevaniformans, Arthrobacter ilicis, Escherichia coli, Pantoea agglomerans, Pseudomonas putida, Pseudomonas aeruginosa, and Micrococcus luteus have also been implicated in phosphate solubilization [11-14].
Microbial inoculants are employed as an alternative source because they are affordable and environmentally beneficial. Although several bacterial and fungal species have been implicated in the solubilization of phosphate, optimal conditions for effective solubilization vary. In addition, it is vital to continue investigating the natural biodiversity of soil microbes and optimize microbial interactions in the rhizosphere. This study, therefore, aimed at screening indigenous bacteria from rhizospheres of selected leguminous and non-leguminous plants for phosphate solubilization potential and determining their optimal conditions for enhanced solubilization under laboratory conditions.
2. MATERIALS AND METHOD
2.1. Bacterial Species
The bacterial strains were isolated from rhizospheres of soybean, cowpea, groundnut, and rice plants, using the standard pour plating procedure. After isolation, representative colonies were streaked on nutrient agar plates to obtain distinct colonies. Distinct colonies were further streaked to ascertain their purity before storing on agar slants and stored at 4 oC ± 2 oC until needed.
Following isolation, a total of 52 bacterial strains were isolated from the rhizospheres and screened for phosphate solubilization in solid and liquid media. The four strains, two species of Enterobacter cloacae and two species of Enterobacter hormaechei, which showed remarkable phosphate solubilization potential, were used for further study.
2.2. Screening for Phosphate Solubilization
Preliminary screening for phosphate solubilization was carried out using Pikovskaya media (glucose 10 g/L, yeast extract 0.5 g/L, ammonium sulphate 0.5 g/L, magnesium sulphate 0.1 g/L, calcium phosphate 0.2 g/L, manganese sulphate 0.002 g/L, ferrous sulphate o.002 g/L, and agar powder 15 g/L), as described elsewhere [15]. Following preparation and sterilization of the media, a 20 mL quantity was dispensed into sterile Petri dishes and allowed to solidify before inoculating with the respective isolates. The inoculated plates were incubated at 35 ± 2 oC for 5 d duration and observed daily for the development of halo zone around each colony. Phosphate solubilization was calculated as follows:
![]() |
Further screening for phosphate solubilization was carried out in Pikovskaya’s broth. To a 250 mL capacity Erlenmeyer flask, containing 150 mL of sterile Pikovskaya’s broth, 1 mL of 18 h broth culture of the respective isolates was inoculated and incubated at 35 ± 2 oC for 5 d. Every 24 h, 5 mL samples were withdrawn from the flasks for estimation of phosphate concentration, using the ascorbic acid method [16].
2.3. Identification of Isolates
The bacterial strains were identified using the 16S rRNA polymerase chain reaction (PCR). For extraction of DNA broth, cultures of the respective bacterial strains were centrifuged at 4600 g for 5 min. The resulting pellets were resuspended in 520 μl of TE buffer (10 mM TrisHCl and 1mM EDTA; pH 8.0). This was followed by the addition of 15 μL of 20% SDS and 3 μl of Proteinase K (20 mg/mL), and incubation at 37 ºC for 1 h. After incubation, 100 μl of 5 M NaCl and 80 μL of 10% CTAB solution in 0.7 M NaCl were added and vortexed, followed by incubation for 10 min at 65 ºC and keeping the solution on ice for 15 min. An equal volume of chloroform:isoamyl alcohol (24:1) was added, followed by incubation on ice for 5 min and centrifugation at 7200g for 20 min. The aqueous phase was then transferred to a new tube and isopropanol (1: 0.6) was added, and DNA was then precipitated at –20 ºC for 16 h. DNA was collected by centrifugation at 13000g for 10 min, washed with 500 μl of 70% ethanol, air dried at room temperature for approximately three hours, and finally dissolved in 50 μl of TE buffer (Frank et al., 2008; Tamura et al., 2013).
The PCR sequencing preparation cocktail used consisted of 10 µl of 5x GoTaq colourless reaction, 3 µl of 25mM MgCl2, 1 µl of 10 mM of dNTPs mix, 1 µl of 10 pmol of each 27F 5‘AGA GTT TGA TCM TGG CTC AG3’ and 1525R, 5‘AAGGAGGTGATCCAGCC3’ primers and 0.3 units of Taq DNA polymerase (Promega, USA) made up to 42 µl with sterile distilled water and 8μl DNA template. The PCR was carried out in a GeneAmp 9700 PCR System Thermal Cycler (Applied Biosystem Inc., USA). The PCR profile consisted of an initial denaturation at 94 °C for 5 min, followed by 30 cycles that consisted of 94 °C for 30 s, 50 °C for 60 s, and 72 °C for 90 s, and a final termination at 72 °C for 10 min.
The integrity of the amplified 1.5Mb gene fragment was checked on a 1% agarose gel to confirm amplification. The gel was electrophoresed at 120V for 45 min, visualized by ultraviolet transillumination, and photographed. The sizes of the PCR products were estimated by comparison with the mobility of a 100bp molecular weight ladder that was run alongside the experimental samples in the gel.
After the determination of the integrity of the gel, the amplified fragments were purified using ethanol to remove the PCR reagents. The purified fragment was checked on a 1.5% agarose gel run at a voltage of 110V for about 1hr as previously to confirm the presence of the purified product and quantified using a nanodrop model 2000 obtained from Thermo Scientific.
The amplified fragments were sequenced using a Genetic Analyzer 3130xl sequencer from Applied Biosystems using the manufacturers’ manual while the sequencing kit used was BigDye Terminator v3.1 cycle sequencing kit. Bio Edit software and MEGA 6 were used for all genetic analyses.
2.4. Optimization of the Parameters for Phosphate Solubilization
Five parameters (carbon source, nitrogen source, incubation temperature, pH, and initial inoculum concentration) were investigated. Sodium acetate, glucose, sucrose, and fructose were used as respective carbon sources, while the nitrogen sources used were ammonium sulphate, peptone, tryptone, and yeast extract. Following the preparation and sterilization of Pikovskaya broth containing the respective carbon or nitrogen sources and inoculation with the respective isolates, the phosphate concentration was estimated every 24 h until the expiration of the incubation period.
To assess the effect of incubation temperature, 25°C, 35°C and 45°C temperatures were used for the investigation using the optimum sodium acetate and ammonium sulphate as carbon and nitrogen sources, respectively. Phosphate concentration in the medium following the inoculation was estimated as described earlier.
To determine the effect of pH, pH 5, pH 7, and pH 9 were used. Following the preparation of the medium, the pH was adjusted using 1 M HCl and NaOH. After adjustment of pH, the medium was sterilized and inoculated. Phosphate concentration was estimated as described earlier.
To assess the effect of inoculum concentration, 1 mL, 2 mL, and 3 mL of 18h old broth cultures of the respective isolates were used. Prior to sterilization, the medium was adjusted to pH 7, while the carbon and nitrogen sources used were sodium acetate and ammonium sulphate, respectively. Following inoculation with the respective isolates, the inoculated flasks were incubated at 35 ± 2 oC. Phosphate concentration was estimated as described earlier.
All experimental analyses were carried out in duplicates.
2.5. Statistical Analysis
The SPSS statistical software (version 23.0) was used for statistical analysis. All comparisons of means were carried out using the one-way analysis of variance test (ANOVA). Multiple comparisons were carried out using the Tukey’s multiple range test. All analyses were carried out at a 95% confidence interval.
3. RESULTS
3.1. Bacterial Strains
Following sequencing, the bacterial strains were identified, as shown in Table 1. The sequences were deposited in the National Centre for Biotechnological Information (NCBI) database, and accession numbers were obtained.
Bacterial Strain | Max Score | Total Score |
Query Cover |
E-value |
% Identity |
Accession |
---|---|---|---|---|---|---|
Enterobacter hormaechei | 2145 | 2145 | 100% | 0 | 100.00% | OP023806 |
Enterobacter cloacae | 2085 | 2085 | 100% | 0 | 97.81% | OP023807 |
Enterobacter cloacae | 2085 | 2085 | 100% | 0 | 97.81% | OP023808 |
Enterobacter hormaechei | 2145 | 2145 | 100% | 0 | 100.00% | OP023810 |
3.2. Effect of Carbon Source
Generally, in media inoculated with the bacterial species, phosphate concentration was not observed to follow any consistent pattern of increase or decrease with the period of incubation. This observation was irrespective of the bacterial isolates or external carbon source in the media. Moreover, although varying phosphate concentrations were observed in the respective media with the carbon sources, these differences did not differ significantly (p≤ 0.05).
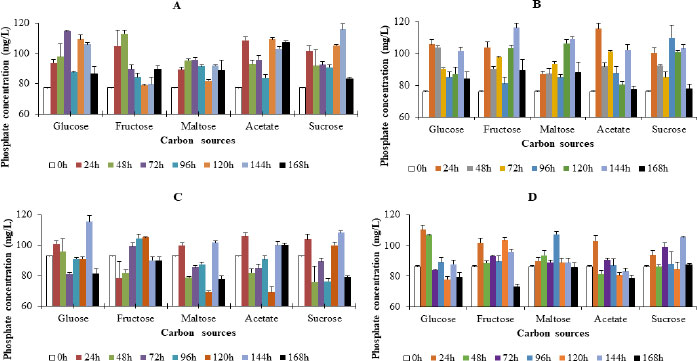
In media inoculated with the Enterobacter cloacae OP023807, the highest phosphate concentrations were observed at 72 h in media that contained glucose (114.58 mg/L) or maltose (95.81 mg/L), while the highest concentration of phosphate of 113.65 mg/L was detected at 48 h of incubation in media that contained fructose. In the case of media with acetate or sucrose, the highest concentrations of 103.12 mg/L (acetate) and 115.94 (sucrose) were, however, detected at a 144-h incubation period (Fig. 1).
For media inoculated with Enterobacter cloacae OP023808, the highest phosphate concentration was observed at 24 h in media containing glucose (105.92 mg/L), fructose (104.03 mg/L), or sodium acetate (115.55 mg/L). The highest phosphate concentrations were observed at 96 and 144 h in media that contained sucrose (110.03 mg/L) and maltose (109.29 mg/L), respectively (Fig. 1).
Moreover, for the media inoculated with Enterobacter hormaechei OP023806, phosphate concentration showed the highest values after 144 h incubation in media that contained glucose (115.50 mg/L), maltose (101.76 mg/L), and sucrose (108.24 mg/L), respectively. In media that contained fructose, the highest phosphate concentration of 105.35 mg/L was recorded at 120 h (Fig. 1).
In the presence of Enterobacter hormaechei OP023810, the highest phosphate concentrations were observed at 24 h incubation in media that contained glucose (110.47 mg/L) or acetate (103.7 mg/L), while 120 h incubation showed the highest phosphate concentration in media with fructose (103.55 mg/L) as the carbon source. In media with maltose or sucrose, the highest concentrations of 107.14 mg/L (maltose) and 105.26 mg/L (sucrose) were, however, detected at 96 h and 144 h incubation periods, respectively (Fig. 1).
3.3. Effect of Nitrogen Source
In the presence of the bacterial species, phosphate concentration did not follow any particular trend throughout the period of incubation. Moreover, no significant differences (p≤ 0.05) were observed between the concentrations of phosphate in media with the respective nitrogen sources. These observations were irrespective of the nitrogen source in the media.
After the 168 h incubation period, the phosphate concentration in the presence of Enterobacter cloacae OP023807 varied from an initial concentration of 111.74 mg/L to concentrations of 103.51 mg/L, 105.30 mg/L, 110.07 mg/L, 105.00 mg/L, and 110.510 mg/L, respectively, in media that contained ammonium sulphate (AS), yeast extract (YE), peptone (P), tryptone (T) and a combination of ammonium sulphate and yeast extract (A+Y), respectively. Generally, the highest phosphate values were recorded at 168 h in media that contained either AS, P or A+Y. In media that contained T or YE, the highest concentration was, however, recorded after 72 h of incubation. The trend in phosphate concentration in the media was observed to be AS > T > YE > A+Y > P (Fig. 2).
In the presence of Enterobacter cloacae OP023808, the phosphate concentration varied from an initial level of 97.34 mg/L to final values of 117.25 mg/L, 110.25 mg/L, 97.83 mg/L, 103.68 mg/L, and 102.20 mg/L, respectively, at the end of incubation in media containing ammonium sulphate, yeast extract, peptone, tryptone, and A+Y, respectively. The highest phosphate values were recorded at 144 h in media that contained ammonium sulphate, at 24 h for peptone, and at 48 h for A+Y. In media that contained tryptone or yeast extract, the highest concentration was, however, recorded after 96h or 168h of incubation. The pattern of phosphate concentration in the media was seen to be YE >A > A+Y > P > T (Fig. 2).
Phosphate levels in media inoculated with Enterobacter hormaechei OP023806 showed the highest concentrations of 102.32 mg/L (120 h), 106.57 mg/L, and 110.86 mg/L (168 h), when ammonium sulphate, A+Y, and peptone were used as nitrogen sources, respectively. In media that contained yeast extract and tryptone as nitrogen sources, the highest phosphate concentrations of 105.74 mg/L and 104.12 mg/L were observed at 144 h, respectively. The pattern of phosphate concentration in the media was YE > A > P > A+Y > T (Fig. 2).
After the 168 h incubation period, the phosphate concentration in the presence of Enterobacter hormaechei OP023810 varied from a concentration of 109.20 mg/L to final values of 98.82 mg/L, 110.9 mg/L, 103.25 mg/L, 101.45 mg/L, and 110.47 mg/L, in media that contained ammonium sulphate, yeast extract, peptone, tryptone, and a combination of ammonium sulphate and yeast extract (A±Y), respectively. Generally, the highest phosphate values were recorded at 168 h in media containing ammonium sulphate as a nitrogen source. In media that contained tryptone or yeast extract, the highest concentration was, however, recorded after 72h of incubation, while in media containing ammonium sulphate or peptone, the highest concentration was recorded after 24h of the incubation period (Fig. 2).
3.4. Effect of Incubation Temperature and pH
No significant difference was observed in phosphate concentrations at different incubation temperatures (p< 0.05). This was irrespective of the test isolate used for inoculation. In media inoculated with Enterobacter cloacae OP023807, isolated from cowpea rhizosphere, the highest phosphate concentrations of 111.87 mg/L, 104.82 mg/L, and 106.44 mg/L were observed after 24 h of incubation in media at 25 oC, 35 oC and 45 oC, respectively. The pattern of phosphate concentration in the media at the respective incubation temperatures was 35 oC > 45 oC > 25 oC (Fig. 3).
For media inoculated with Enterobacter cloacae OP023808, phosphate concentration showed the highest increase at 24 h of incubation. This was irrespective of the incubation temperatures used for the investigation. The highest phosphate concentrations of 121.02 mg/L, 109.98 mg/L, and 117.34 mg/L were observed at incubation temperatures of 25 oC, 35 oC, and 45 oC, respectively (Fig. 4). Generally, the average phosphate concentration during incubation was 45 oC> 35 oC > 25 oC (Fig. 3).
In the case of media inoculated with Enterobacter hormaechei OP023806, the highest phosphate concentrations of 99.66 mg/L, 221.15 mg/L, and 118.52 mg/L were observed at 24 h of incubation in media at 25 oC, 35 oC and 45 oC, respectively (Fig. 4). The pattern of average phosphate concentration in the media was 25 0C > 45 oC > 35 oC (Fig. 3). In media inoculated with the Enterobacter hormaechei OP023810, the highest phosphate concentrations of 111.60 mg/L (25 oC), 111.86 mg/L (35 oC), and 115.28 mg/L (45oC), were observed at 24 h of incubation. The pattern of phosphate concentration in the media was 45 oC< 25 oC < 35 oC (Fig. 3).
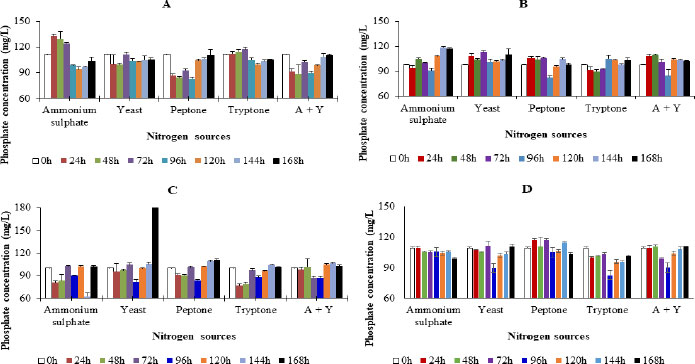
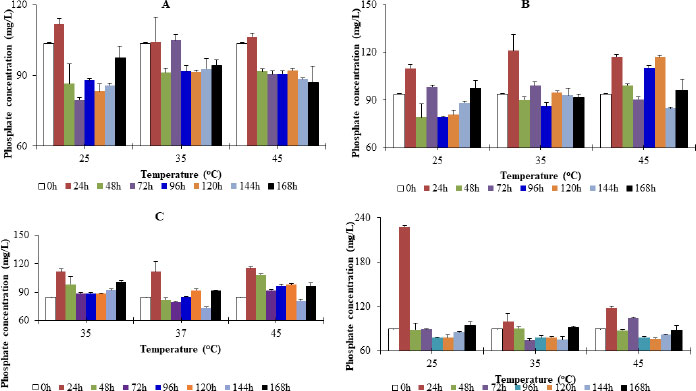
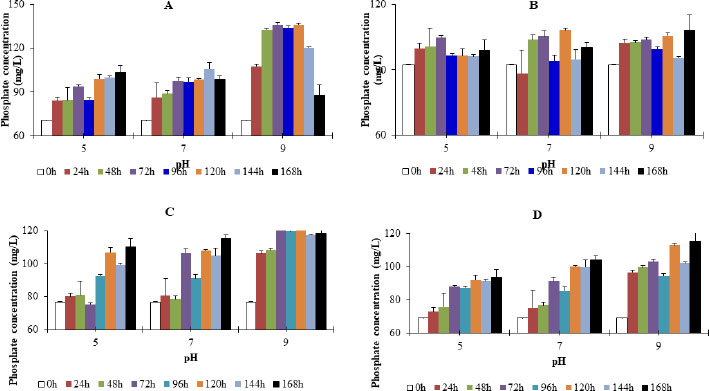
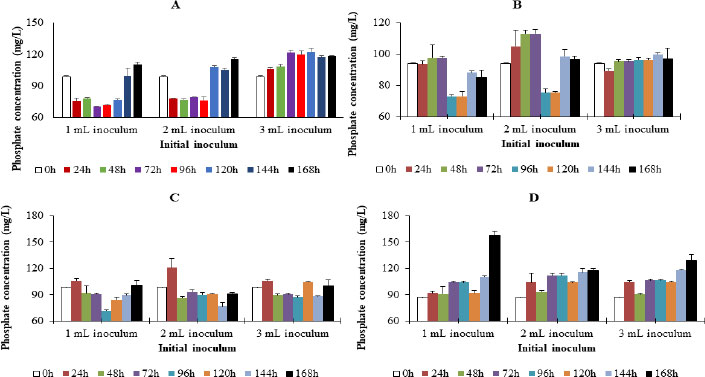
In the presence of all bacterial species, the phosphate concentration in the media at the respective pH was observed to be pH 9> pH7> pH 5. This observation was made irrespective of the test isolate used for inoculation. When the media was inoculated with the Enterobacter cloacae OP023807, the phosphate concentration in the media at pH 9 was observed to be significantly higher than in media at all other pH (Fig. 4).
For media inoculated with Enterobacter cloacae OP023808, although phosphate concentration increased with an increase in pH of the media, the differences in concentration at different pH did not differ significantly (Fig. 4).
In the case of media inoculated with Enterobacter hormaechei OP023806, although phosphate concentration should have increased with higher pH in the media, these differences did not occur significantly (Fig. 4).
For media inoculated with the Enterobacter hormaechei OP023810, although a higher phosphate concentration was observed at pH 9, this was only observed to be significantly higher than media at pH 5 and not pH 7. There was no significant difference observed in phosphate levels in the media at pH 7 and 5 (Fig. 4).
3.5. Effect of Initial Inoculum Concentration
In media inoculated with Enterobacter cloacae OP023807, phosphate concentration was observed to increase with the increase in inoculum concentration. The average phosphate concentration in the media was observed to be 3 mL inoculum> 2 mL inoculum> 1 mL inoculum. The concentration of phosphate in the presence of 3mL inoculum was observed to be significantly higher than that of 1mL inoculum (Fig. 5).
The trend in phosphate concentration in media inoculated with Enterobacter cloacae OP023808 was 2 mL inoculum > 3 mL inoculum> 1 mL inoculum. Differences in phosphate concentration in the media in the presence of the respective initial inoculum did not differ significantly (Fig. 5).
When inoculated with the respective initial inoculum of Enterobacter hormaechei OP023806 in the media, the phosphate concentration during incubation was 3 mL inoculum > 2 mL inoculum > 1 mL inoculum. However, the difference in the phosphate concentration did not differ significantly (Fig. 5).
Moreover, when inoculated with Enterobacter hormaechei OP023810, the trend in phosphate level in the media was 3mL inoculum > 2mL inoculum > 1mL inoculum. The differences in phosphate concentration did not, however, differ significantly (Fig. 5).
4. DISCUSSION
The present study revealed no visible trend in the phosphate concentration of the respective carbon sources. However, the highest phosphate concentrations were observed in media that contained either glucose or acetate as an external carbon source. Glucose has been reported as an ideal carbon source for phosphate solubilization [17]. Some authors [18] have reported higher phosphate solubilization by Bacillus sp. NPSBS 3.2.2 in the presence of sucrose as a carbon source. Moreover, Lebrazi et al. [19] have reported maltose to be an ideal carbon source for phosphate release in the soil in the presence of Aspergillus awamori S19 isolated from the rhizospheric soil. In this study, all carbon sources investigated enhanced phosphate solubilization in the presence of the bacterial species. This supports the findings of Chen and Liu [17], who found Enterobacter sp. to have the maximum effectiveness for phosphate solubilization when glucose was used as the growing medium. According to a related study by Nautiyal et al. [20] on stress-induced phosphate solubilization in bacteria isolated from alkaline soils, all four of the bacteria species under investigation displayed varied degrees of phosphate solubilization in the presence of the various carbon sources used. The isolate NBRI0603 was found to be incapable of solubilizing phosphate effectively in a medium containing sucrose, sorbitol, maltose, or lactose as the only carbon source, according to the study. The secretion of phosphatase, an enzyme responsible for the transformation of the complex and unavailable forms of phosphorous into a soluble and assimilable one, was found to be higher when Aspergillus caespitosus was incubated with polysaccharides. However, intracellular secretion was observed when it was incubated with glucose or disaccharides [21].
In this study, the highest phosphate concentration was reported in media that contained ammonium sulphate. It is indicated that nitrogen source has a significant effect on increasing the amount of soluble phosphorus. Lebrazi et al. [19] have reported the trend in phosphate solubilization as ammonium nitrate > sodium nitrate > tryptone > peptone > urea. This study's findings corroborate the observation of Rezakhani et al. [22]. Ammonium and nitrate salts were found to be efficient nitrogen sources during microbial phosphate solubilization in a study by Liu et al. [23]. According to reports, the presence of ammonium in a growth media causes inorganic acids to form through a proton exchange mechanism [24]. However, if the medium becomes strongly acidic, it can have a counter-effect. This is because phosphorus can easily form complexes with Fe and Al under acidic conditions. The availability of nitrogen makes it easy for microbes to build up their body cells and, hence, channel their investment of nitrogen to the production of phosphatase [25]. Conversely, a high availability of phosphatase boosts the activity of phosphatase and, consequently, phosphorus solubility.
Additionally, it is suggested that the presence of ammonium can cause organisms that solubilize phosphate to excrete organic acid metabolites [26]. The investigation also showed xylose, sucrose, and sorbitol to be poor carbon sources for the solubilization of phosphate by isolating NBRI4003, whereas sucrose and sorbitol were reported to be poor carbon sources for NBRI3246. For phosphate solubilisation in strains NBRI0603, NBRI2601, NBRI3246, and NBRI4003, xylose, lactose, xylose, and glucose were reported as the best carbon sources, respectively. The isolates showed outstanding phosphate solubilization of all nitrogen sources tested in this study. However, the current investigation found ammonium sulphate-containing media to have the highest levels of phosphate solubilization. A study conducted by Osorio and Habte [27] reported a similar observation. As reported by Shekhar et al., phosphate solubilization by NBRI0603, NBRI2601, NBRI3246, and NBRI4003 changed when ammonium and various sources of nitrates were utilized as nitrogen sources [28]. The positive effect of yeast extract on boosting phosphate solubilization by tested isolates could be explained by the fact that yeast extract serves as both a source of growth factors and a nitrogen supply, both of which are crucial for promoting microbial growth and phosphate solubilization.
The best pH and temperature in this investigation were found to be 9 and 35 °C, respectively, enabling the test isolates' ability to dissolve phosphate. These findings are in line with those reported by Nopparat et al. [29], who found the optimum pH and temperature for the bacterial strain under study to solubilize phosphate as 8.84 and 34.7 °C, respectively. Mardad et al. [30] reported that Enterobacter hormaechei produced the most orthophosphate at pH 7 and 30 °C in a related investigation. Additionally, Yi et al. [31] reported that solubilized phosphate of 111.7 mgP/L was found when the culture media's pH was decreased from 7.0 to 4.5 when Aeromonas sp. was present. When the culture medium's pH dropped from 7.0 to 6.0 in the presence of Enterobacter sp., the study found solubilized phosphate of 632.6 mgP/L. It is opined that phosphate solubilization is not only dependent on culture medium pH, but also on other factors, such as exopolysaccharide production by microorganisms [20]. This can be attributed to the larger amount of exopolysaccharide produced by Enterobacter sp. One of the most crucial factors affecting the synthesis of enzymes and other bioprocesses, like phosphate solubilizing activity, is temperature. Rodrguez and Fraga [32] made a similar observation, stating that Enterobacter sp., in general, has emerged as a very efficient phosphate solubilizer under a wide range of pH, salt, and temperature, as reflected by its high phosphate solubilization efficiency, and that these isolates grow well at elevated salt level, pH, and temperature; thus, they may be suitable for the development of potential bio-inoculants for soils containing insoluble phosphorus.
The investigation generally showed maximum phosphate concentrations in various setups and optimization circumstances after 72 h of incubation. This observation held true regardless of the isolates utilized in the study. Maximum phosphate solubilization was noted to occur at the end of the logarithmic phase in a related work by Walpola and Arunakumara [33]. According to the study, at days 2 and 3 of incubation, respectively, the maximum levels of phosphate solubilisation occurred when Enterobacter ludwigii and Enterobacter hormaechei were present. Additionally, in the study by Jena and Rath [23], the optimal incubation period for phosphate solubilizing activity of the bacterial isolates they studied was discovered to be 3 days. Another study found Rhizobium leguminosarum to consistently increase the amount of phosphate it could dissolve the longer it was incubated [34].
CONCLUSION
The aim of this research was to optimize the solubility of phosphorus in selected bacteria strains and, hence, its availability to plants. Generally, it was found that the ability of the selected bacteria strains to increase the solubility of phosphorus depends on other factors, like carbon and nitrogen sources, pH, temperature, and duration of incubation. The extent of phosphate solubilization of the test strains was not observed to be dependent on the external carbon sources investigated. The best nitrogen source was found to be ammonium sulphate and the highest solubility was attained at a pH of 9, temperature of 35 ◦C, and incubation period of 72 h. The outcome of this research is valuable in the field of agronomy and environmental sciences. The unavailability of phosphorus because of its complex nature can be reduced and it can be made available to plants with the introduction of these bacterial strains.
LIST OF ABBREVIATIONS
NCBI | = National Centre for Biotechnological Information. |
ANOVA | = one-way analysis of variance test. |
ETHICS APPROVAL AND CONSENT TO PARTICIPATE
Not applicable.
HUMAN AND ANIMAL RIGHTS
Not applicable.
AVAILABILITY OF DATA AND MATERIALS
Not applicable.
CONSENT FOR PUBLICATION
Not applicable.
FUNDING
None.
CONFLICT OF INTEREST
The authors declare no conflict of interest, financial or otherwise.
ACKNOWLEDGEMENTS
The authors are grateful to Afe Babalola University for providing the facilities for this study.