All published articles of this journal are available on ScienceDirect.
Network of Interaction among Enterobacter Species and Klebsiella Pneumonia Clinical Isolates and the Antibiotic Resistance Pattern at Cape Coast
Abstract
Introduction:
Urgent surveillance is required to provide the needed information for the containment of multidrug-resistant (MDR) Enterobacteriaceae. The objective was to examine the antibiotic-resistant patterns among the clinically isolated Enterobacter species and Klebsiella pneumonia from the outpatient department of the University of Cape Coast from 2014 to 2020.
Methodology:
The cultured isolates were Gram-stained for morphological and biochemical tests. Antibiotic susceptibility tests for the K. pneumonia and Enterobacter spp were done using the modified Kirby-Bauer diffusion technique. A double-disk synergy antibiotic test using cefotaxime, amoxicillin/clavulanic acid and ceftazidime antibiotic test was used to identify extended-spectrum beta-lactase (ESBL) and MDR among the isolates. The network of interactions between bacteria isolates and resistant types and the pattern of interaction of K. pneumonia (in the resistant-10 group) and Enterobacter spp (resistant-12) and the various antibiotics tested was performed using Cytoscape version 3.8.2 software. The data are presented in percentage and statistical significance between MDR and extensively drug-resistant (XDR) compared with Chi-square test.
Results:
K. pneumonia formed a clustering network with other bacteria isolates around resistant type 10 (resistant-10) and Enterobacter spp around resistant type 12 (resistant-12). The interactions (edges) of bacteria isolates and resistant types (nodes) were unique for Staphylococcus saprophyticus with Proteus mirabilis which exhibited two patterns of interactions (resistant-10 and resistant-12), connecting the two resistant types among the bacteria isolates. The interactive network patterns of Enterobacter spp and the various antibiotics were different from the interaction patterns for K. pneumonia. The K. pneumonia isolates were highly resistant to cefuroxime 2/3 (66.67%) and ampicillin 2/3 (66.67%) in the year 2020. Enterobacter spp on the other hand were highly resistant to tetracycline 16/20 (80.00%) in 2014 and ceftriaxone 5/9 (55.56%), levofloxacin 5/9 (55.56%), ceftazidime 5/9 (55.56%) and gentamycin 5/9 (55.56%) in 2020. Chi-square analysis showed a highly significant difference of χ2 = 11.87, p=0.0006 and χ2 = 7.789, p=0.0053 between MDR and XDR Enterobacter spp isolates in 2014 and 2020, respectively.
Conclusion:
Early detection, constant monitoring and control practices and policies that prevent the misuse or overuse of these antibiotics are required to slow down the rapid development of resistance to Enterobacter spp and K. pneumonia in Cape Coast.
1. INTRODUCTION
The emergence of multiple antibiotic-resistant Enterobacteriaceae is a global threat to health and security [1, 2]. The increased resistance of Enterobacteriaceae to fluoroquinolones, aminoglycosides, carbapenems, monobactam, cephalosporins and extended-spectrum penicillins limits the current antibiotic treatment options [3, 4]. The Enterobacter species and Klebsiella pneumonia are the most frequently reported pathogenic Enterobacteriaceae cases in community-acquired and nosocomial infections [5-7]. These pathogens cause a wide range of human infections including uropathogenic complications [8, 9].
Extended-spectrum β-lactamases (ESBLs) Enterobacter spp and K. pneumonia infections have become a serious clinical issue in many global communities including Ghana [10-14]. The high morbidity and mortality associated with pathogenic bacteria infections have been linked to the extensive emergence of antibiotic resistance [15, 16]. This trend is, however, alarming as the resistance mechanisms have been associated with AmpC, blaCTX-M, blaTEM and blaSHV genes carried by the pathogens [17, 18]. Most of these mediate resistance through plasmid transfer between pathogens [19]. Thus, antibiotic-sensitive pathogens can acquire resistance by obtaining resistance-encoded genes without antibiotic exposure [20]. The extensive antibiotic resistance among clinically isolated Enterobacter spp and K. pneumonia complicates the choice of appropriate antibiotics for treatment [21-23].
Antibiotic resistance (AMR) has been predicted to be the greatest problem facing low and middle-income countries [24-27]. However, antibiotic stewardship and appropriate policy direction on antibiotic usage to prevent complete antibiotic failure is hindered by limited data on the aetiology and susceptibility patterns of clinical isolates from these countries [24, 26]. The spreading of carbapenem-resistant Enterobacteriaceae (CRE) threatens the treatment of multidrug-resistant (MDR) Enterobacteriaceae with the latest carbapenems such as meropenem, imipenem, and ertapenem [28-30]. Currently, there is no new antimicrobial agent to forestall the unsatisfactory management of CRE [31, 32]. This requires urgent surveillance in low- and middle-income countries to provide the needed data for the containment of AMR [25, 27].
A recent study on ESBL-producing Enterobacteriaceae in Ghana reported a 49.3% prevalence with 17% being resistant to two or more aminoglycosides, fluoroquinolones, sulfonamide, and carbapenems antibiotics and 3.2% non-ESBL producers [33]. A similar study in other parts of Ghana had also reported 25.9% of blaTEM, 28.8% blaSHV, 26.6% blaCTX-M, 2.16% blaOXA-48 and 0.72% blaNDM among Klebsiella spp with ESBL, AmpC β-lactamase (AmpC), and carbapenemase resistance [34]. Little is known about the Enterobacter spp isolated from clinical samples and their antibiotic resistance mechanisms [35]. Currently, there is no study in Ghana that has analyzed the annual trends and prevalence of antibiotic resistance among clinical isolates of Enterobacter spp and K. pneumonia infections. The objective, was to examine the antibiotic-resistant patterns among clinically isolated Enterobacter spp and K. pneumonia from the outpatient department of the University of Cape Coast hospital from 2014 to 2020.
2. MATERIALS AND METHODS
2.1. Study Area
K. pneumoniae and Enterobacter spp isolate from 2014 to 2020 at the University of Cape Coast hospital in the Central region of Ghana were evaluated for their antibiotic resistance patterns. The University hospital provides health services to students, University staff and the surrounding communities. The hospital is about 160 meters from the shores of the Gulf of Guinea and is located close to the main entrance of the University campus.
2.2. Bacteria Isolation and Identification of Pathogens
Patients referred to the Microbiology Laboratory for diagnosis and isolation of bacterial infection had their samples cultured using Blood agar (Sigma-Aldrich) and MacConkey agar (Sigma-Aldrich) and incubated at 37°C overnight. The morphological characteristics of the bacteria isolates were performed using macroscopic and microscopic Gram staining examinations. Biochemical identification of bacteria isolates was performed by culturing the isolates on Endo agar (Sigma-Aldrich), MacConkey broth (Sigma-Aldrich), and Simmons citrate agar (Sigma-Aldrich) selective culture media. The isolates were characterized using catalase, coagulase, oxidase, sugar fermentation, indole, citrate utilization, urease production, and motility tests with E. coli O157 as a control.
2.3. Antibiotic Susceptibility Test (AST)
Antibiotic susceptibility test (AST) was performed using Mueller-Hinton agar antibiotic diffusion technique (Kirby-Bauer NCCLS modified disc diffusion technique) as previously described [36]. The pure bacteria colonies were emulsified in normal saline and compared with 0.5 McFarland solution. Ampicillin, Gentamicin, Cotrimoxazole, Cefuroxime, Erythromycin, Amikacin, amoxicillin/clavulanic acid, cefuroxime, cefotaxime, ceftazidime, imipenem, Cefixime, Cefotaxime, Penicillin, Cloxacillin, ertapenem, meropenem, tetracycline, trimethoprim-sulfamethoxazole, gentamicin, nalidixic acid, ciprofloxacin, chloramphenicol, aztreonam, piperacillin/tazobactam, fosfomycin, and colistin antibiotic susceptibilities were tested.
2.4. Detection and Confirmation of Extended-spectrum Beta-lactamase (ESBL)
ESBLs resistance K. pneumoniae and Enterobacter spp isolates were diagnosed using the double-disk synergy antibiotic susceptibility test of Cefotaxime (CTX), Amoxicillin/Clavulanic acid (AMC) and Ceftazidime (CAZ) antibiotic discs. The isolates were cultured on a Mueller Hinton agar plate with appropriate antimicrobial-impregnated disks at 35oC overnight. The inhibition zones were measured from the circumference to the distinct edges from the centre of inhibitions using a rule. The level of ESBL resistance by K. pneumoniae and Enterobacter spp were calculated using the formula (inhibition zone values * 2). The E. coli ATCC 25922 strains were used as a control.
2.5. Statistical Analysis
The data was validated and analyzed using Excel 2016 (Microsoft Corporation) and GraphPad Prism 9.0.2 software. The bacteria isolates were classified based on the median antibiotic inhibition zones into resistant type 0 (resistant-0, no antibiotic susceptibility), resistant-8, resistant-9, resistant-10, resistant-11 and resistant-12 (the predominant median antibiotic inhibition zones were 8, 9, 10, 11 and 12 mm, respectively for most of the antibiotic tested). A network of interactions between bacteria isolates and resistant types and the pattern of interaction of K. pneumonia (in the resistant-10 group) and the various antibiotics tested; was performed using Cytoscape version 3.8.2 software. The annual pattern of ESBLs and MDRs among K. pneumoniae and Enterobacter spp were tested using the Chi-square test with p<0.05 considered as statistically significant.
3. RESULTS
3.1. Demographic Characteristics of the Study Subjects
In all, 230 subjects that visited the University of Cape Coast hospital had Enterobacter spp. and/or K. pneumonia bacteria isolated from their clinical samples from January 2014 to April 2020. The median (range) age/years of the subjects were 25 (5-73), 27 (1-60), 33 (5-83), 32 (10-85), 26 (10-73), 29 (3-90) and 24 (1-70) for 2014, 2015, 2016, 2017, 2018, 2019 and 2020, respectively. The majority of the subjects were females 197/230 (85.65%) and males representing 33/230 (14.35%). Most of the pathogens were isolated from urine samples followed by high vaginal swabs (HVS) samples. Overall, Enterobacter spp was the most isolated pathogen 132/230 (57.39%) followed by K. pneumonia 98/230 (42.61%) (Table 1).
3.2. Pattern and Network of Interactions between Antibiotic Resistance and Bacteria Isolates from 2014 to 2020
The bacteria isolates from 2014 to 2020 at the University of Cape Coast hospital were classified into resistant types (resistant-0, resistant-8, resistant-9, resistant-10, resistant-11 and resistant-12) based on the most predominant median antibiotic inhibition zone of resistance. The result showed a clustering network of bacteria isolates around resistant type 10 (resistant-10) and resistant type 12 (resistant-12) (Table S1). The interactions (edges) of bacteria isolates and resistant types (nodes) were unique for Staphylococcus saprophyticus, and Proteus mirabilis which exhibited two patterns of interactions (resistant-10 and resistant-12), connecting the two resistant types among the bacteria isolates. The other resistant types formed smaller clusters of bacteria isolates (Fig. 1).
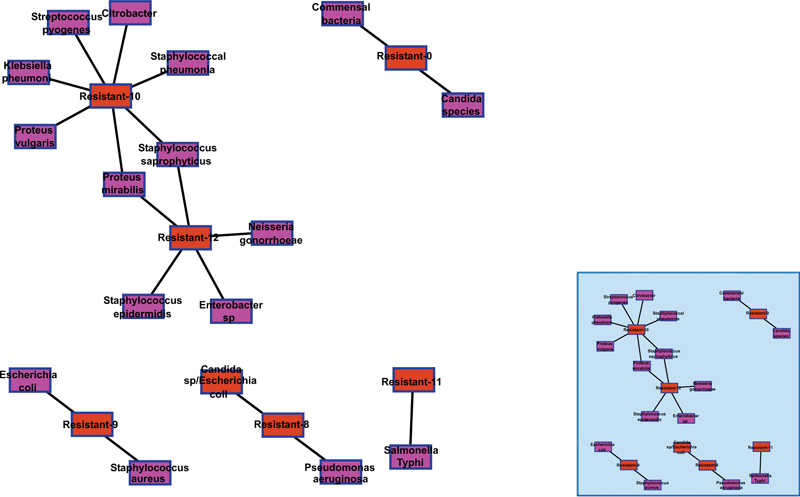
Table 1.
- | - | - | - | Years | - | - | - |
---|---|---|---|---|---|---|---|
Parameters | 2014 | 2015 | 2016 | 2017 | 2018 | 2019 | 2020 |
Isolates, N | 27 | 24 | 41 | 49 | 26 | 51 | 12 |
Age, median (range)/yrs | 25 (5-73) | 27 (1-60) | 33 (5-83) | 32 (10-85) | 26 (10-73) | 29 (3-90) | 24 (1-70) |
Sex, n (F/M) | 27 (24/3) | 24 (17/7) | 41 (39/2) | 49 (41/8) | 26 (22/4) | 51 (43/8) | 12 (11/1) |
Sample Types, n/N (%) | - | - | - | - | - | - | - |
HVS | 4/27 (14.81) |
6/24 (25.00) |
10/41 (24.39) |
17/49 (34.70) |
9/26 (34.62) |
16/51 (31.37) |
7/12 (58.33) |
Urine | 22/27 (81.49) |
12/24 (50.00) |
31/41 (74.61) |
32/49 (65.30) |
17/26 (65.38) |
35/51 (68.63) |
5/12 (41.67) |
Sputum/Ear | 1/27 (3.70) |
3/24 (12.50) |
- | - | - | - | - |
Wound | - | 3/24 (12.50) |
- | - | - | - | - |
Bacteria, n/N (%) | - | - | - | - | - | - | - |
Enterobacter species | 20/27 (74.07) |
7/24 (29.17) |
19/41 (46.34) |
22/49 (44.90) |
16/26 (61.38) |
39/51 (76.47) |
9/12 (75.00) |
Klebsiella pneumonia | 7/27 (25.93) |
17/24 (70.83) |
22/41 (53.66) |
27/49 (55.10) |
10/26 (38.46) |
12/51 (23.53) |
3/12 (25.00) |
3.3. Klebsiella pneumonia and Enterobacter species Resistance and Antibiotic Interaction Network
The interaction network between K. pneumonia (resistant-10) isolates and antibiotics tested showed that the K. pneumonia isolates had developed a broader resistance to multiple antibiotics; fluoroquinolones, aminoglycosides, carbapenems, monobactams, cephalosporins and extended-spectrum penicillins. Few K. pneumonia isolates formed lower clusters of one, two, three and four antibiotic types (Fig. 2). The interactive network patterns of Enterobacter spp (resistant-12) and the various antibiotics were different from the interaction patterns for K. pneumonia (Fig. 3).
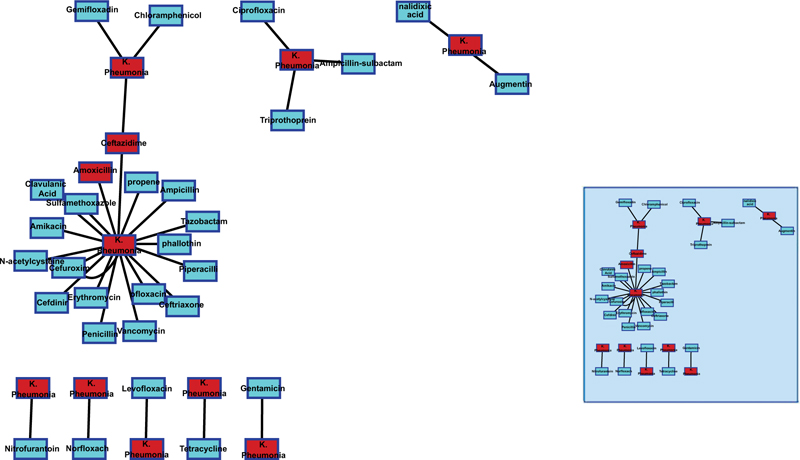
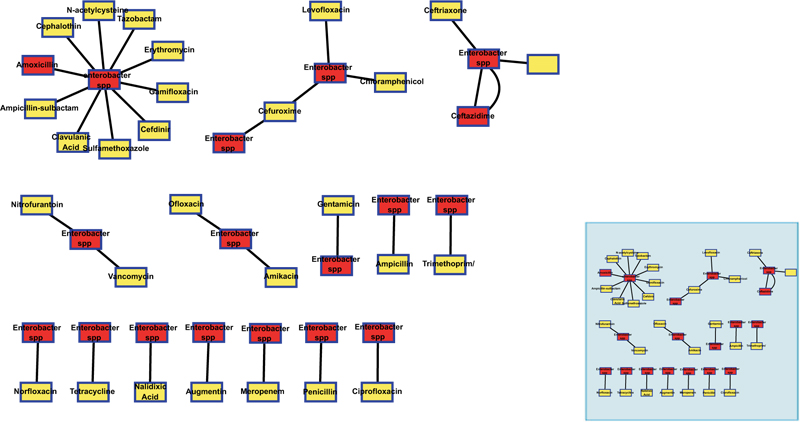
3.4. Multiple Drug Resistance (MDR) and Extended Drug Resistance (XDR) Patterns among Enterobacter Species and Klebsiella Pneumonia Bacteria Isolates
The pattern of XDR resistance among the K. pneumonia isolates is constantly increasing from 2/7 (28.57%) in 2014 to 9/12 (75.00%) in 2019 and 2/3 (66.67%) in 2020. The MDR resistance among K. pneumonia, on the other hand, has remained high from 2014 to 2018, with the highest MDR resistance of 3/4 (75.00%) observed in 2018. In 2019 and 2020, K. pneumonia exhibited high XDR resistance compared to MDR resistance. However, the chi-square analysis did not show any significant differences in the emergence of K. pneumonia resistance to MDR and XDR. In the case of Enterobacter spp (resistant-12), MDR resistance was very high 19/20 (95.00%) compared to 1/20 (5.00%) of the XDR resistance isolates in 2014. However, from 2015 to 2020, most Enterobacter spp exhibited resistance to XDR compared to MDR. Chi-square analysis showed a highly significant difference χ2 = 11.87, p=0.0006 between MDR and XDR Enterobacter spp isolates in 2014. Although more of the Enterobacter spp isolates were resistant to XDR, there was no significant difference between MDR and XDR except in 2019, where XDR resistance Enterobacter spp isolates were significantly different from MDR resistance Enterobacter spp isolates χ2 = 7.789, p=0.0053 (Table 2).
- | Klebsiella pneumonia n/N (%) | - | Enterobacter species n/N (%) | - | ||||
---|---|---|---|---|---|---|---|---|
Year | MDR | XDR | χ2 | p | MDR | XDR | χ2 | p |
2014 | 5/7 (71.43) |
2/7 (28.57) |
0.875 | 0.3496 | 19/20 (95.00) |
1/20 (5.00) |
11.87 | 0.0006 |
2015 | 10/17 (58.82) |
7/17 (41.18) |
0.354 | 0.5518 | 3/7 (42.86) |
4/7 (57.14) |
0.095 | 0.7574 |
2016 | 12/22 (54.55) |
10/22 (45.45) |
0.121 | 0.7276 | 9/19 (47.37) |
10/19 (52.63) |
0.035 | 0.8514 |
2017 | 15/27 (55.56) |
12/27 (44.44) |
0.222 | 0.6371 | 12/22 (54.55) |
10/22 (45.45) |
0.121 | 0.7276 |
2018 | 3/4 (75.00) |
1/4 (25.00) |
0.685 | 0.4076 | 6/9 (66.67) |
3/9 (33.33) |
0.675 | 0.4113 |
2019 | 3/12 (25.00) |
9/12 (75.00) |
2.057 | 0.1515 | 9/39 (23.08) |
30/39 (76.92) |
7.789 | 0.0053 |
2020 | 1/3 (33.33) |
2/3 (66.67) |
0.225 | 0.6353 | 3/9 (33.33) |
6/9 (66.67) |
0.675 | 0.4113 |
- | - | - | - | - | Antibiotic Resistance n (%) | - | - | - | - | - | ||||
---|---|---|---|---|---|---|---|---|---|---|---|---|---|---|
- | 2014 | 2015 | 2016 | 2017 | 2018 | 2019 | 2020 | - | ||||||
Antibiotics | Klebsiella pneumonia (n=7) | Enterobacter sp (n=20) | Klebsiella pneumonia (n=17) | Enterobacter sp (n=7) | Klebsiella pneumonia (n=22) | Enterobacter sp (n=19) | Klebsiella pneumonia (n=27) | Enterobacter sp (n=22) | Klebsiella pneumonia (n=4)$ | Enterobacter sp (n=9)* | Klebsiella pneumonia (n=12) | Enterobacter sp (n=39) | Klebsiella pneumonia (n=3) | Enterobacter sp (n=9) |
Ceftriaxone | 1 (14.29) | - | 3 (17.65) | - | - | 3 (15.79) | 6 (22.22) | 3 (13.64) | 1 (25.00) | 1 (11.11) | 1 (8.33) | 11 (28.21) | 1 (33.33) | 5 (55.56) |
Levofloxacin | 3 (42.6) | 3 (15.00) | - | - | 3 (13.64) | 4 (21.05) | 7 (25.93) | 3 (13.64) | - | - | 1 (8.33) | 9 (23.08) | - | - |
Ceftazidime | 2 (28.57) | - | 4 (23.53) | 3 (42.6) | 2 (9.09) | 2 (10.53) | 6 (22.22) | 3 (13.64) | - | 1 (11.11) | 4 (33.33) | 14 (35.90) | 1 (33.33) | 5 (55.56) |
Ciprofloxacin | 2 (28.57) | 7 (35.00) | 1 (5.88) | 1 (14.29) | 3 (13.64) | 3(15.79) | 2 (7.41) | - | - | 1 (11.11) | 2 (16.67) | 18 (46.15) | - | 5 (55.56) |
Gentamicin | 1 (14.29) | 8 (40.00) | 5 (29.41) | 1 (14.29) | 6 (27.27) | 6 (31.58) | 9 (33.33) | 13 (59.09) | - | - | 6 (50.00) | 9 (23.08) | - | - |
Cefuroxime | 1 (14.26) | - | 5 (29.41) | - | 5 (22.73) | 3 (15.79) | 7 (25.93) | 3 (13.64) | - | 2 (22.22) | - | 13 (33.33) | 2 (66,67) | 4 (44.44) |
Chloramphenicol | 4 (57.14) | 1 (5.00) | 5 (29.41) | 4 (57.14) | 1 (4.55) | 3 (15.79) | 7 (25.93) | 5 (22.73) | - | 1 (11.11) | 1 (8.33) | 5 (12.82) | - | 1 (11.11) |
Ampicillin | 1 (14.29) | - | 8 (47.06) | 4 (57.14) | 7 (31.82) | 2 (10.53) | 14 (51.85) | 7 (31.82) | - | 3 (33.33) | 4 (33.33) | 17 (43.59) | 2 (66,67) | 5 (55.56) |
Trimethoprim/ | 2 (28.57) | 7 (35.00) | 8 (47.06) | 3 (42.6) | 6 (27.27) | 4 (21.05) | 13 (48.15) | 7 (31.82) | 3 (33.33) | 3 (25.00) | 10 (25.64) | 3 (33.33) | ||
Sulfamethoxazole | ||||||||||||||
Nitrofurantoin | 1 (14.29) | 9 (45.00) | 1 (5.88) | 1 (14.29) | 3 (13.64) | 2 (10.53) | 9 (33.33) | 8 (36.36) | 2 (50.00) | 2 (22.22) | 3 (25.00) | 5 (12.82) | - | 2 (22.22) |
Amoxicillin/ | 1 (14.29) | 2 (10.53) | 2 (50.00) | 2 (22.22) | 1 (11.11) | |||||||||
Clavulanic Acid | ||||||||||||||
Piperacillin/ | 1 (14.29) | 13 (59.09) | 11 (57.89) | 6 (22.22) | 7 (31.82) | 1 (25.00) | 5 (55.56) | 3 (25.00) | 10 (25.64) | 1 (11.11) | ||||
Tazobactam | ||||||||||||||
Cefuroxime | 1 (14.29) | - | 1 (5.88) | - | 6 (27.27) | 4 (21.05) | 8 (29.63) | 8 (36.36) | 1 (25.00) | 1 (11.11) | 5 (41.67) | 13 (33.33) | 1 (33.33) | 2 (22.22) |
Norfloxacin | 1 (14.29) | 2 (10.00) | 1 (5.88) | 1 (14.29) | 4 (18.18) | 4 (21.05) | 4 (14.81) | 6 (27.27) | - | - | 2 (16.67) | 12 (30.77) | - | - |
Tetracycline | 3 (42.6) | 16 (80.00) | 9 (52.94) | 6 (85.71) | 18 (81.82) | 15 (78.95) | 19 (70.37) | 15 (68.18) | - | 7 (77.78) | 4 (33.33) | 26 (66.67) | 1 (33.33) | 3 (33.33) |
Nalidixic Acid | 1 (14.29) | 11 (55.00) | - | 1 (14.29) | 11 (50.00) | 10 (52.63) | 5 (18.52) | 8 (36.36) | - | 1 (11.11) | 4 (33.33) | 13 (33.33) | 1 (33.33) | - |
Augmentin | 1 (14.29) | 11 (55.00) | 4 (23.53) | 3 (42.6) | 14 (63.64) | 11 (57.89) | 10 (37.04) | 12 (54.55) | 3 (75.00) | 4 (44.44) | 6 (50.00) | 16 (41.03) | - | 2 (22.22) |
Gemifloxacin | 1 (14.29) | 1 (5.00) | - | - | - | - | - | - | - | 1 (11.11) | - | - | - | 1 (11.11) |
Ampicillin-sulbactam | 1 (14.29) | 7 (35.00) | - | - | - | - | - | - | - | - | - | - | - | - |
Ceftazidime | - | 1 (5.00) | 3 (17.65) | 1 (14.29) | 8 (36.36) | 8 (42.11) | 6 (22.22) | 7 (31.82) | 1 (25.00) | 2 (22.22) | 5 (41.67) | 16 (41.03) | 1 (33.33) | 1 (11.11) |
Meropenem | - | - | 4 (23.53) | 3 (42.6) | 5 (22.73) | 5 (26.32) | 8 (29.63) | 4 (18.18) | - | 2 (22.22) | 3 (25.00) | 11 (28.21) | 1 (33.33) | 4 (44.44) |
Vancomycin | - | - | 5 (29.41) | 2 (28.57) | 7 (31.82) | 4 (21.05) | 9 (33.33) | 3 (13.64) | - | 3 (33.33) | 2 (16.67) | 8 (20.51) | 1 (33.33) | 1 (11.11) |
N-acetylcysteine | - | - | - | - | - | 1 (5.26) | - | - | - | 1 (11.11) | - | - | - | - |
Cefdinir | - | - | - | - | - | 1 (5.26) | - | - | - | - | - | - | - | - |
Cephalothin | - | - | - | - | - | 3 (15.79) | - | 3 (13.64) | - | 1 (11.11) | - | 3 (7.69) | - | - |
Penicillin | - | - | - | 1 (14.29) | 1(4.55) | - | 1 (3.70) | - | - | - | - | - | - | - |
Erythromycin | - | - | - | 1 (14.29) | 1 (4.55) | - | - | - | - | - | - | - | - | - |
Ofloxacin | - | - | - | - | - | - | 1 (3.70) | 2 (9.09) | - | - | - | - | - | - |
Amikacin | - | - | - | - | - | - | 1 (3.70) | - | - | - | - | 1 (2.56) | - | - |
* Antibiotic susceptibility test carried on 9 out of 16 Enterobacter species isolates.
3.5. Antibiotic Resistance Pattern among Enterobacter species and Klebsiella pneumonia Isolates
K. pneumonia isolates were highly resistant to levofoxacin 3/7 (42.6%), chloramphenicol 4/7 (57.14%), and tetracycline 3/7 (42.6%) in 2014. The K. pneumonia isolates were observed to have developed resistance to many of the commonly used antibiotics in the market from 2015 to 2017 and 2019. K. pneumonia resistance to tetracycline 9/17 (52.94%) in 2015; piperacillin/tazobactam 13/22 (59.09%), tetracycline 18/22 (81.82%), nalidixic acid 11/22 (50.00%), augmentin 14/22 (63.64%) in 2016; ampicillin 14/27 (51.85%), trimethoprim/sulfamethoxazole 13/27 (48.15%), tetracycline 19/27 (70.37%) in 2017; nitrofurantoin 2/4 (50.00%), amoxicillin/clavulanic acid 2/4 (50.00%) and augmentin 3/4 (75.00%) in 2018; gentamycin 6/12 (50.00%), and augmentin 6/12 (50.00%) in 2019 and cefuroxime 2/3 (66.67%) and ampicillin 2/3 (66.67%) were very high across the study periods (Table 3).
On the other hand, Enterobacter spp were highly resistant to tetracycline 16/20 (80.00%), nalidixic acid 11/20 (55.00%), and augmentin 11/20 (55.00%) in 2014; chloramphenicol 4/7 (57.14%), ampicillin 4/7 (57.14%), and tetracycline 6/7 (85.71%) in 2015; piperacillin/tazobactam 11/19 (57.89%), tetracycline 15/19 (78.95%), nalidixic acid 10/19 (52.63%), and augmentin 11/19 (57.89%) in 2016; gentamycin 13/22 (59.09%), and tetracycline 15/22 (68.18%) in 2017; piperacillin/tazobactam 5/9 (55.56%), and tetracycline 7/9 (77.78%) in 2018; tetracycline 26/39 (66.67%) in 2019 and ceftriaxone 5/9 (55.56%), levofloxacin 5/9 (55.56%), ceftazidime 5/9 (55.56%), gentamycin 5/9 (55.56%), and ampicillin 5/9 (55.56%), in 2020 (Table 3).
4. DISCUSSION
Enterobacter spp and K. pneumonia have developed resistance to almost all known antibiotics [37]. There is a report of increasing high resistance to third and fourth-generation cephalosporins [38]. Although ESBL-resistant Enterobacter spp such as E. cloacae and E. aerogenes are generally susceptible to carbapenems, there is growing resistance to most of them except Meropenem and Imipenem [39-41]. Also, carbapenem-resistant Enterobacter (CRE) and carbapenem-resistant K. pneumoniae infections are susceptible to polymyxins, tigecycline, meropenem, fosfomycin, gentamicin, ceftazidime/avibactam and other co-carbapenems antibiotic agents [42, 43]. Antibiotic-resistant Enterobacter spp and K. pneumonia are reducing treatment options leading to “difficult-to-treat” high morbidity and mortality cases [44]. The study reports an eight-year antibiotic resistance trend among the Enterobacter spp and K. pneumonia isolates from the University of Cape Coast hospital.
K. pneumonia formed a network with a group of bacteria isolates that exhibit phenotypically similar antibiotic resistance inhibition patterns (belonging to resistant type 10; median antibiotic inhibition zone is 10), whereas Enterobacter spp formed a clade with other bacteria isolates with resistant type 12. Thus, K. pneumonia and Enterobacter spp phenotypically exhibit differences in antibiotic resistance characteristics [45]. Interestingly, S. saprophyticus and P. mirabilis exhibited characteristics of both resistant-10 and resistant-12. However, resistant type 10 and type 12 are not distinctive in terms of their resistant categorization into MDR or XDR. Both resistant types were either MDR or XDR species. Although both K. pneumonia and Enterobacter spp have developed resistance to several antibiotics, the underlying mechanisms may be multifaceted and different [45]. The interactive network of K. pneumonia or Enterobacter spp and the various antibiotics showed that most antibiotics have similar median and range in the zone of inhibitions.
The pattern of MDR and XDR among the isolates showed the rapid development of XDR. MDR-resistant K. pneumonia was consistently high from 2014 to 2018 while XDR resistance became high in 2019. However, XDR-resistant Enterobacter spp had remained high compared to MDR resistance except in 2014. The high prevalence of XDR K. pneumonia and Enterobacter spp pose a tremendous challenge to treatment options and may require prolonged antibiotic treatment [42, 46]. XDR-resistant Enterobacter spp and K. pneumonia infections should be of public health significance as these resistant pathogens are not only resistant to multiple antibiotic agents but likely all clinically proven antibiotics [47, 48].
MDR and XDR K. pneumonia and Enterobacter spp are a threat to the public health system in Ghana as they are significant risk factors for severe illness with high mortality [49]. The recent outbreak of K. pneumonia producing NDM-1 and OXA-48 in Germany with increased virulence, high transmission and antibiotic resistance indicates global health importance that requires constant surveillance and prompt implementation of enhanced control measures to prevent the spread [50-52].
The study showed that Enterobacter spp and K. pneumonia isolates were highly susceptible to fluoroquinolones (levofloxacin, Norfloxacin, Gemifloxacin and ofloxacin), beta-lactams (ampicillin-sulbactam and amoxicillin-clavulanic acid), aminoglycoside (Amikacin), macrolide (erythromycin), penicillin, N-acetylcysteine, and cephalosporin (cefdinir) antibiotics. Although ampicillin-sulbactam resistance has been recorded in beta-lactamase-producing Enterobacteriaceae such as E. coli, amoxicillin-clavulanic acid remains an effective therapy against such pathogens [53, 54]. Interestingly, these antibiotics were largely effective against the MDR and XDR-resistant K. pneumonia and Enterobacter spp isolates from the University of Cape Coast hospital. Though the isolated MDR and XDR Enterobacter spp and K. pneumonia are still susceptible to the above antibiotics, resistance against these antibiotics can rapidly develop [55-57].
Constant risk assessment of MDR and XDR carbapenem-resistant Enterobacteriaceae and updates on the prevalence of antibiotic resistance patterns are necessary preventive and control measures [56, 58]. Again, meropenem and gentamicin which have shown to be effective against XDR carbapenem-resistant Enterobacteriaceae were highly resisted among the isolated pathogens in this study. The limited treatment options for antibiotic-resistant Enterobacteriaceae necessitated the WHO to declare Enterobacteriaceae a critical and priority pathogen for research, discovery and development of new antibiotics [3, 46, 57]. The state of antibiotic-resistant Enterobacter spp and K. pneumonia requires a nationwide reassessment of antibiotic treatment options.
Two large clusters of bacteria networks of interaction around resistant-10 and resistant-12 (nodes). The resistant types are classified based on the frequencies of median antibiotic inhibition zones; Resistant-0 (no antibiotics were tested), Resistant-8 (most antibiotics had inhibition zone of 8 mm), Resistant-9 (most antibiotics had inhibition zone of 9 mm), Resistant-10 (most antibiotics had inhibition zone of 10 mm), Resistant-11 (most antibiotics had inhibition zone of 11 mm) and Resistant-12 (most antibiotics had inhibition zone of 12 mm).
The length of each node connecting to K. pneumonia represents the median (range) antibiotic inhibition zones for each tested antibiotic.
The length of each node connecting to Enterobacter spp represents the median (range) antibiotic inhibition zones for each tested antibiotic.
Several factors may have affected the female-to-male ratio among the data analyzed; 1. There were more females attending hospitals compared to males, however, the reason is not well understood, but it is believed females take a keen interest in their health compared to males as it is a general trend with most of the healthcare data, 2. The nature of samples for microbiological data was (36.5% were vaginal samples which is a bias toward the female gender, and 63.5% were urine samples 17.5% of which were from males.
CONCLUSION
In conclusion, the study reports the trend of antibiotic resistance among Enterobacter spp and K. pneumonia isolates from the University of Cape Coast hospital from 2014 to 2020. The results show that although there is high MDR and XDR-resistant Enterobacteriaceae, the pathogens are still susceptible to a couple of antibiotics tested. The early detection, constant monitoring and control practices and policies that prevent misuse or overuse of these antibiotics are required to slow down the rapid development of resistant Enterobacter species and Klebsiella pneumonia in Cape Coast.
LIST OF ABBREVIATIONS
MDR | = Multidrug Resistant |
ESBL | = Extended-spectrum beta-lactase |
XDR | = Extensively Drug Resistant |
CRE | = Carbapenem-resistant Enterobacteriaceae |
ETHICS APPROVAL AND CONSENT TO PARTICIPATE
Not applicable.
HUMAN AND ANIMAL RIGHTS
No animals and humans were used for studies that are the basis of this research.
CONSENT FOR PUBLICATION
Not applicable.
AVAILABILITY OF DATA AND MATERIALS
The authors confirm that the data supporting the findings of this study are available within the article.
FUNDING
None.
CONFLICT OF INTEREST
The authors declare no conflict of interest, financial or otherwise.
ACKNOWLEDGEMENTS
Declared none.
SUPPLEMENTARY MATERIALS
Supplementary material is available on the Publisher’s website.