All published articles of this journal are available on ScienceDirect.
Distribution of Leukocidins, Exfoliative Toxins, and Selected Resistance Genes Among Methicillin-resistant and Methicillin-sensitive Staphylococcus aureus Clinical Strains in Egypt
Abstract
Background:
Infection with Staphylococcus aureus (S.aureus) is an increasing health problem worldwide. This pathogen has multiple virulence factors that contribute to its pathogenesis in a wide range of diseases. The present study aimed to investigate the prevalence of leukocidins, exfoliative toxins, and common antimicrobial resistance genes among Methicillin-Resistant Staphylococcus aureus (MRSA) and Methicillin-Sensitive Staphylococcus aureus (MSSA) strains collected from various clinical sources in Egypt.
Methods:
Isolates were identified as S.aureus by the standard microbiological methods. Methicillin resistance was detected phenotypically by cefoxitin disc diffusion method and genotypically by PCR for detection of mecA gene. PCR was also used to detect the presence of leukocidin genes (LukD, LukE, LukF-PV, and LukS-PV), exfoliative toxin genes (eta and etb), and antibiotic resistance genes (tetK, tetM, ermA, ermC, msrA, and aacA-aphD).
Results:
About 50.5% of tested isolates were methicillin resistant by cefoxitin disc assay, while mecA gene was amplified in 64.6% of isolates. The highest prevalent toxin gene was lukE (93%) and the least prevalent one was eta (1%). The resistance genes tetK and tetM were detected in nearly 50% of the tested strains but lower prevalence rates were recorded for aacA-aphD, msrA, ermA, and ermC genes.
Conclusion:
Methicillin resistance was highly prevalent among tested S.aureus strains. Regarding the studied virulence and resistance genes, no significant difference was detected between MRSA and MSSA strains, except for ermA gene p<0.05 which was highly prevalent in MRSA strains. So, the variation between MRSA and MSSA strains in the response to treatment may be attributed to the resistance of MRSA strains to all β-lactams in addition to other possible acquired resistance mechanisms. Accordingly, fewer options of antimicrobial medications are available to treat MRSA infections.
1. INTRODUCTION
Despite being carried in the anterior nares of about 20 - 80% of healthy individuals [1], S.aureus continues to be a major health threat for both humans and animals. This bacterium can cause multiple illnesses that vary in severity from mild self-limiting conditions to serious health complications [2].
The large number of virulence factors associated with S.aureus presents a major obstacle in controlling its detrimental impact on human health. These virulence factors help the spread of infection inside and outside the host through facilitating colonization and invasion processes. They include adhesins, enzymes, immune evading proteins, and toxins [3]. The expression of these virulence factors can be controlled by the surrounding conditions, where expression levels can be up-regulated or down-regulated. During colonization, virulence factors that trigger rapid immune response are down-regulated while those facilitating adhesion and evasion of host immune response are up-regulated [4].
Leukocidins (Luk) and exfoliative toxins (ETs) are among the important toxins secreted by some strains of S.aureus. These toxins have varied damaging activities on host cells. Panton-Valentine leukocidin (PVL), leukocidin ED (LukED), leukocidin AB, γ-haemolysin AB, and γ-haemolysin CB are staphylococcal bi-component pore-forming leukotoxins. The two protein subunits of each toxin act synergistically, resulting in pore formation and cell lysis. The main targets of the staphylococcal leukocidins are host leukocytes [5]. The staphylococcal scalded skin syndrome appears when exfoliative toxin A (ETA) and exfoliative toxin B (ETB) are secreted by the invading S.aureus strains. Both toxins exfoliate the skin epidermis through cleavage of desmoglein-1 which mediates keratinocyte adhesion. Although both toxins are genetically similar and act by the same mechanism, the eta gene encoding ETA toxin is chromosomally-mediated, while etb gene encoding ETB toxin is found on plasmid DNA [6].
In addition to the enormous number of virulence factors, a high level of antimicrobial resistance is also found in most of S.aureus strains. Of special concern is methicillin resistance that constitutes a significant barrier to successful treatment of S.aureus infections. The first report of Methicillin-Resistant Staphylococcus aureus (MRSA) was in 1961. Since then, MRSA infections whether hospital-acquired or community-acquired have been a major health problem in many countries [7]. The penicillin-binding proteins 2a (PBP2a) encoded by mecA gene were found to mediate resistance to methicillin and other β-lactam antibiotics in Staphylococcus species [8]. Besides, resistance mechanisms to other classes of antibiotics were also observed e.g. secretion of degrading enzymes that degrade and inactivate antibiotics. As a result, only limited treatment options are currently available for treatment of MRSA among which vancomycin still comes in the first place [9]. In Egypt, the prevalence rate of MRSA varies by region. MRSA was found in high numbers among ICU patients at Alexandria-University Hospital (75%) and in patients hospitalized at Cairo University Hospitals (47.9%) [10]. In a study by Abdel-Maksoud et al. during the period of 2005 to 2013, MRSA represented 76.6% and 11.5% of hospital-acquired and community-acquired S. aureus isolates, respectively [11].
As a macrolide antibiotic, clindamycin is efficiently used for treatment of MRSA infections. However, with its wide use, many strains became resistant to it [12]. Multidrug-resistant S.aureus is usually treated by a combination of glycopeptide and aminoglycoside drugs. Thus, resistance to aminoglycosides is commonly reported among S.aureus isolates [13]. Few studies in Egypt detected leukocidin genes and exfoliative toxin genes in both MRSA and MSSA strains. So, the present study aimed to investigate the prevalence of these virulence genes in addition to some resistance genes for drugs that are commonly used for treatment of these infections in Egyptian hospitals including selected resistance genes for tetracyclines, aminoglycosides, and macrolides (ermA, ermC, msrA, accA-aphD, tetK, and tetM).
2. MATERIALS AND METHODS
2.1. Collection, Isolation, and Identification of Clinical Specimens
The present study was authorized by the research ethics committee, faculty of pharmacy, Mansoura University, Egypt (Code 2021 - 237). A total of 99 clinical S.aureus strains were isolated from different clinical specimens (exudates from abscesses, burns, wounds, boils, diabetic foot infections, vaginal infections, sputum, and urine). They were collected from patients admitted to four major governmental hospitals in Mansoura city, Egypt from April 2017 to February 2018. The specimens were aseptically transferred to the Microbiology and Immunology Lab, faculty of pharmacy, Mansoura University for culturing, isolation, and identification of bacterial isolates. Each specimen corresponded to a single isolate. Cultures were grown on mannitol salt agar and were subjected to further analysis via Gram staining, culture on blood agar to demonstrate hemolysis, catalase, DNase, and coagulase tests [14].
2.2. Detection of Methicillin Resistance
Kirby-Bauer disc diffusion method was used for phenotypic detection of methicillin resistance in S.aureus strains using cefoxitin discs (FOX, 30 µg) and Muller Hinton agar medium (Oxoid). The test was performed and the results were interpreted as MRSA or MSSA according to the 2018 Clinical and Laboratory Standards Institute (CLSI) guidelines [15]. Genotypic detection of methicillin resistance was confirmed by detection of mecA gene using mecA primers listed in Table 1 [16-21].
Gene | Primer sequence | Annealing temperature (°C) | Amplicon size (bp) | References |
eta | Fw GCAGGTGTTGATTTAGCATT | 57 | 93 | [16] |
Rw AGATGTCCCTATTTTTGCTG | ||||
etb | Fw ACGGCTATATACATTCAATT | 50 | 200 | |
Rw TCCATCGATAATATACCTAA | ||||
LukS | Fw GGTCCATCAACAGGAGGTAAT | 57 | 267 | [17] |
Rw AGGATTGAAACCACTGTGTACT | ||||
Luk E | Fw TGCGTAAATACCAGTTCTAGGG | 60 | 199 | |
Rw TCCAACAGGTTCAGCAAGAG | ||||
LukD | Fw ACCAGCATTTGAACTACTTTGT | 60 | 240 | |
Rw TCTAATGGCTTATCAGGTGGAT | ||||
Luk F | Fw TGTGCTTCTACTTTCCACCAT | 54 | 225 | |
Rw TGTGACTGACTTTGCACCA | ||||
mecA | Fw GAGTTGAACCTGGTGAAGTTGTAATC | 60 | 335 | [18] |
Rw ATGAAGATGGCTATCGTGTCACA | ||||
tetK | Fw GTAGCGACAATAGGTAATAGT | 51 | 360 | [19] |
Rw GTAGTGACAATAAACCTCCTA | ||||
tetM | Fw AGTGGAGCGATTACAGAA | 51 | 158 | |
Rw CATATGTCCTGGCGTGTCTA | ||||
aacA-aphD | Fw TACAGAGCCTTGGGAAGATG | 54 | 406 | [20] |
Rw CATTTGTGGCATTATCATCATATC | ||||
ermA | Fw TATCTTATCGTTGAGAAGGGATT | 54 | 139 | [21] |
Rw CTACACTTGGCTGATGAAA | ||||
ermC | Fw AATCGTCAATTCCTGCATGT | 54 | 299 | [19] |
Rw TAATCGTGGAATACGGGTTTG | ||||
msrA | Fw TGCAAATGGCATACTATCGTC | 56 | 160 | [20] |
Rw CAAGAACGCTCAAGTGCTTC |
2.3. Genotypic Detection of Leukocidin, Exfoliative Toxin, and Antibiotic Resistance Genes
2.3.1. Preparation of Genomic DNA
The tested strains were streaked on nutrient agar plates. Following incubation at 37°C for 18-24 h, a single colony of each strain was picked up and homogenized in 0.1 ml of DNase/RNase free water, heated in a thermocycler at 95°C for 10 min, chilled on ice and then centrifuged. The supernatants were then transferred to sterile Eppendorf tubes and kept at -20°C to be used as DNA templates in PCR [22].
2.3.2. Polymerase Chain Reaction for Detection of the Tested Genes
Polymerase chain reaction was used to detect the presence of leukocidin genes (LukD, LukE, LukF-PV, and LukS-PV), exfoliative toxin genes (eta and etb), and antibiotic resistance genes; (tetK, tetM, ermA, ermC, msrA, and aacA -aphD). Primers were obtained from Invitrogen. In a PCR tube, 12.5 μl Dream Taq™ Green PCR Master Mix (2X) (Thermo scientific), 1 μl of forward primer (10 μM), 1 μl of reverse primer (10 μM), 5 μl of the DNA template, and 5.5 μl of nuclease-free water were mixed gently, placed in a thermocycler to amplify tested genes. The following cycling conditions were used: Denaturation at 94 °C for 5 min, 35 cycles; denaturation at 95°C for 30 sec, annealing at the appropriate annealing temperature (Table 1) for 30 sec, and extension at 72°C for 40 sec; and a final extension at 72°C for 5 min. Negative controls were included in all reactions while positive controls were available only for eta and etb genes using DMS 19048 standard strain (provided by Dr. Paolo Moroni, University of Milan, Italy). The PCR products were separated by electrophoresis on a 1.5% agarose gel stained with ethidium bromide and visualized using a gel documentation system. List of primer sequences, annealing temperatures, and expected amplicons sizes are shown in Table 1.
2.4. Statistical Analysis
The data presented in this study were analyzed by Chi-square test to compare the MRSA and MSSA groups and the correlation between the studied genes was also evaluated by Spearman rank correlation coefficient test using SPSS (version 13; SPSS Inc.) software. The difference is considered statistically significant at P value of less than 0.05.
3. RESULTS
3.1. Collection, Isolation, and Identification of Clinical Specimens
In the current study, ninety-nine bacterial isolates were identified as S.aureus based on their positive reactions in catalase, DNase, and coagulase tests, beside appearing as Gram-positive cocci in Gram staining.
3.2. Detection of Methicillin Resistance
Cefoxitin disc diffusion method was used for phenotypic screening of methicillin resistance. Referring to CLSI 2018, 50.5% of S.aureus strains were reported as MRSA. Genotypic detection of methicillin resistance through mecA gene was investigated as well. The result showed that 64 S.aureus strains harbored mecA gene but 16 (25%) of them were sensitive to methicillin. Concurrently, 5.7% of mecA negative strains showed resistance to methicillin (Table 2).
Table 2.
Detection of methicillin resistance | Genotypically (mecA gene) | |||
Present | Absent | Total | ||
Phenotypically (cefoxitin) | Resistant | 48 | 2 | 50 |
Sensitive | 16 | 33 | 49 | |
Total | 64 | 35 | 99 |
3.3. Genotypic Detection of Leukocidin, Exfoliative Toxin, and Antibiotic Resistance Genes
Fig. (1) shows the prevalence of leukocidin and exfoliative toxin genes in tested S.aureus strains. The most abundant leukocidin gene was lukE (93.9%) followed by lukD (62.6%), lukF-PV (56.6%), and lukS-PV (15.1%). The lukE-lukD gene combination was prevalent in 62 strains (62.6%) while lukSF-PV gene combination was present in 8 strains. The least prevalent genes were eta (1%) and etb (5.1%). Based on Spearman’s rank correlation coefficient test, a significant positive correlation was found between lukD, lukE, and lukF genes.
Selected resistance genes for tetracyclines, aminoglycosides, and macrolides were also detected. tetK and tetM genes were present in nearly half of the tested strains. The prevalence rates of aacA-aphD, msrA, ermA, and ermC were 32.3%, 44.4%, 17.2%, and 41.4%, respectively (Fig. 2). The distribution of toxin and resistance genes among MRSA and MSSA strains and its statistical significance are indicated in Table 3. A positive significant correlation was detected between the following gene pairs; lukD/tetK, lukD/tetM, lukD/aacA-aphD, lukF/tetK, tetK/tetM, and msrA/aacA-aphD (P< 0.05, 0.2 < r < 0.5).
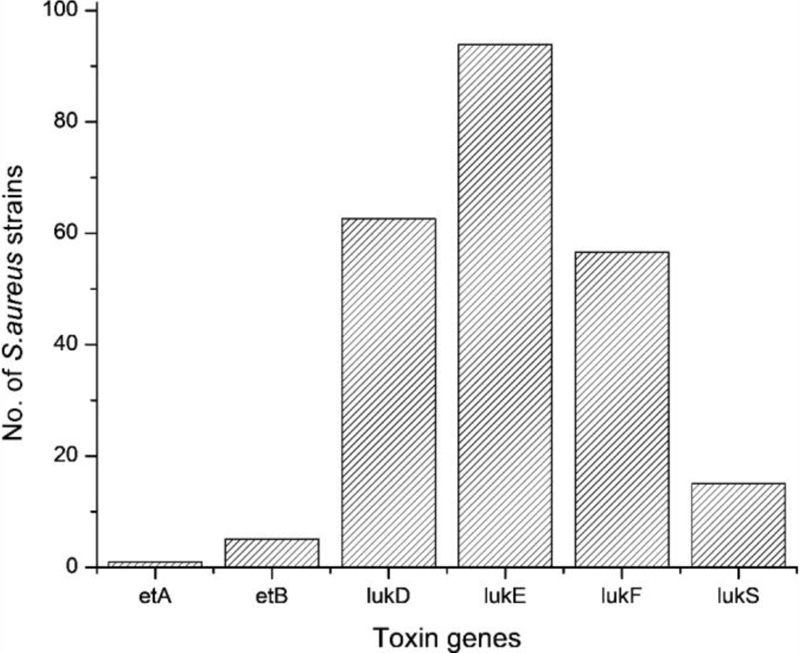
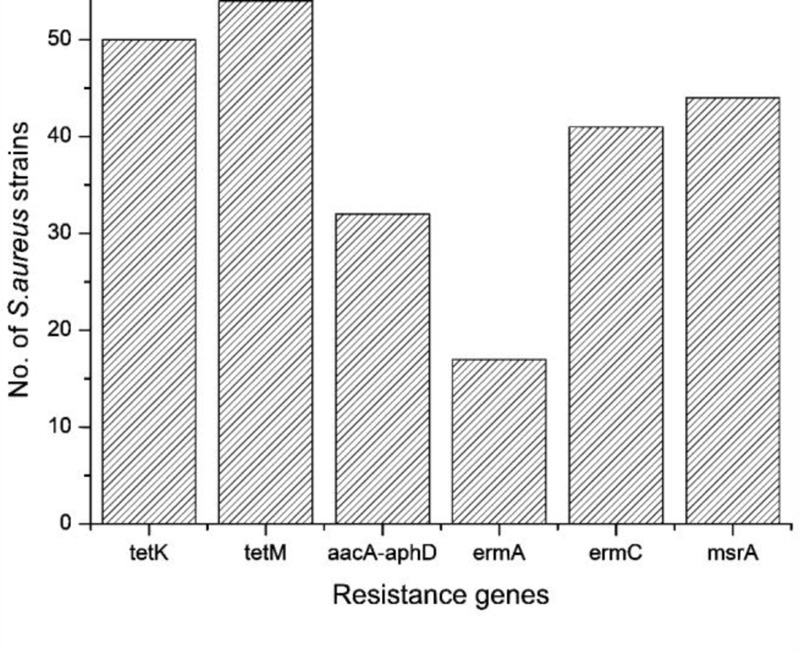
Gene | No. of strains (%) | |||
MRSA (64 strain) | MSSA (35 strain) | Total | P- value | |
eta | 1 (1.6) | 0 (0) | 1 | 0.457 |
etb | 4 (6.3) | 1 (2.9) | 5 | 0.461 |
lukD | 42 (65.6) | 20 (57.1) | 62 | 0.404 |
lukE | 60 (93.8) | 33 (94.3) | 93 | 0.915 |
lukF-PV | 38 (59.4) | 18 (51.4) | 56 | 0.446 |
LukS-PV | 11 (17.2) | 4 (11.4) | 15 | 0.445 |
LukSF-PV | 6(9.3) | 2(5.7) | 8 | 0.523 |
tetK | 33 (51.6) | 17 (48.6) | 50 | 0.776 |
tetM | 35 (54.7) | 19 (54.3) | 54 | 0.969 |
aacA-aphD | 25 (39.1) | 7 (20.0) | 32 | 0.053 |
ermA | 16 (25.0) | 1 (2.9) | 17 | 0.025* |
ermC | 26 (40.6) | 15 (42.9) | 41 | 0.829 |
msrA | 28 (43.8) | 16 (45.7) | 44 | 0.851 |
By analyzing toxin and resistance genes distribution patterns, S.aureus strains were classified into thirty-eight resistance genotypes (R1-R38) and fourteen toxin genotypes (T1-T14) based on the coexistence pattern of the selected resistance and toxin genes in tested isolates. As for resistance, 13 profiles were common between MRSA and MSSA strains, 21 profiles were found in MRSA strains only while 4 profiles appeared in MSSA only (Table 4). For toxin genes, 14 profiles were presented in MRSA strains but only 9 of them were detected in MSSA strains, with T10 predominating in 40% of S.aureus strains (Table 5).
Resistance genotype | Resistance genotype profile | No. of positive MRSA strains | No. of positive MSSA strains | Total No. of positive S.aureus strains |
R1 | No genes detected | 4 | 5 | 9 |
R2 | tetM | 4 | 0 | 4 |
R3 | tetK | 1 | 2 | 3 |
R4 | ermA | 2 | 0 | 2 |
R5 | ermC | 3 | 1 | 4 |
R6 | msrA | 2 | 0 | 2 |
R7 | aacA-aphD | 2 | 2 | 4 |
R8 | tetK, tetM | 5 | 3 | 8 |
R9 | tetM, ermC | 0 | 1 | 1 |
R10 | tetM, ermA | 1 | 1 | 2 |
R11 | tetM, msrA | 1 | 3 | 4 |
R12 | ermC, msrA | 2 | 3 | 5 |
R13 | ermA, ermC | 1 | 0 | 1 |
R14 | aacA-aphD, msrA | 3 | 0 | 3 |
R15 | aacA-aphD, ermA | 1 | 0 | 1 |
R16 | tetK, tetM, ermA | 1 | 0 | 1 |
R17 | tetK, tetM, ermC | 5 | 2 | 7 |
R18 | tetK, ermA, msrA | 1 | 0 | 1 |
R19 | tetM, ermC, msrA | 0 | 1 | 1 |
R20 | tetK, tetM, msrA | 2 | 3 | 5 |
R21 | tetM, ermA, ermC | 0 | 1 | 1 |
R22 | tetK, tetM, aacA-aphD | 1 | 0 | 1 |
R23 | aacA-aphD, ermA, msrA | 1 | 0 | 1 |
R24 | tetM, aacA-aphD, msrA | 1 | 0 | 1 |
R25 | tetK, aacA-aphD, msrA | 1 | 1 | 2 |
R26 | tetK, aacA-aphD, ermA | 1 | 0 | 1 |
R27 | aacA-aphD, ermC, msrA | 1 | 0 | 1 |
R28 | tetK, tetM, ermA, ermC | 1 | 0 | 1 |
R29 | tetK, tetM, ermC, msrA | 3 | 2 | 5 |
R30 | tetK, tetM, aacA-aphD, ermC | 2 | 1 | 3 |
R31 | tetK, tetM, aacA-aphD, msrA | 2 | 0 | 2 |
R32 | tetK, aacA-aphD, ermC, msrA | 2 | 2 | 4 |
R33 | aacA-aphD, ermA, ermC, msrA | 1 | 0 | 1 |
R34 | tetM, aacA-aphD, ermC, msrA | 1 | 0 | 1 |
R35 | tetK, tetM, aacA-aphD, ermC, msrA | 1 | 1 | 2 |
R36 | tetK, tetM, aacA-aphD, ermA, msrA | 1 | 0 | 1 |
R37 | tetK, tetM, aacA-aphD, ermA, ermC | 1 | 0 | 1 |
R38 | tetK, tetM, aacA-aphD, ermA, ermC, msrA | 2 | 0 | 2 |
Toxin genotype | Toxin genotype profile | No. of positive MRSA strains | No. of positive MSSA strains | Total No. of positive S.aureus strains |
T1 | No toxins | 3 | 1 | 4 |
T2 | lukE | 11 | 9 | 20 |
T3 | etb | 1 | 1 | 2 |
T4 | etb, lukE | 1 | 0 | 1 |
T5 | lukS, lukE | 2 | 1 | 3 |
T6 | lukD, lukE | 5 | 4 | 9 |
T7 | lukE, lukF | 3 | 3 | 6 |
T8 | lukS, lukD, lukE | 2 | 1 | 3 |
T9 | lukS, lukE, lukF | 1 | 0 | 1 |
T10 | lukD, lukE, lukF | 27 | 13 | 40 |
T11 | etb, lukS, lukD, lukE | 1 | 0 | 1 |
T12 | etb, lukD, lukE, lukF | 1 | 0 | 1 |
T13 | eta, lukD, lukE, lukF | 1 | 0 | 1 |
T14 | lukS, lukD, lukE, lukF | 5 | 2 | 7 |
4. DISCUSSION
S.aureus is a major life-threatening pathogen that is highly prevalent worldwide. The main challenge in treating S.aureus infections is the increasing resistance of this bacterium to antimicrobial agents beside its high virulence capacity.
In the present study, ninety-nine S.aureus strains were isolated from different clinical specimens which demonstrates the wide diversity of S.aureus infections. Methicillin resistance was detected by phenotypic and genotypic methods in 50.5% and 64.6% of S.aureus strains, respectively. This finding is in agreement with previous reports, which recorded high rates of methicillin resistance in Lebanon, Iran, China, and Egypt [11, 18, 23]. In contrast to the present study, lower rates of methicillin resistance were reported in Nigeria [9], USA [24], and Denmark [25]. As shown in Table 2, 16 strains that were sensitive to methicillin by cefoxitin disc diffusion method harbored mecA gene that codes for methicillin resistance. This can be explained by the heterogeneous expression of methicillin resistance which can be altered by growth conditions such as pH, temperature, and inoculum size. Consequently, mecA gene detection provides a more accurate way for detection of methicillin resistance rather than the cefoxitin disc diffusion assay. Among mecA negative S.aureus strains, 2 strains showed phenotypic resistance to methicillin. This finding coincides with previous studies that detected methicillin resistance in mecA negative isolates of S.aureus [26, 27]. A study conducted in Sudan suggested that methicillin resistance may be attributed to other intrinsic factors, which may explain phenotypic resistance to methicillin in mecA negative isolates [28].
Leukocidins are cytolytic toxins produced by some strains of S.aureus. In this study, lukE gene was prevalent in 93.9% of strains followed by lukD (62.6%), lukF-PV (56.6%), and lukS-PV (15.1%) genes. All strains having lukD gene were found to have lukE gene. This gene combination encodes the two protein subunits of LukED toxin that act in synergy to lyse cells. In addition, the lukSF-PV gene combination was detected in eight strains (8.1%); this combination encodes the powerful PVL toxin, and was more prevalent in MRSA strains (9%) than in MSSA strains (5%). Previous studies had observed more prevalence of PVL genes among MRSA strains isolated from skin and soft tissue infections than MSSA strains [29, 30]. In contrast to this result, Sila et al. reported a higher prevalence of PVL genes in MSSA strains compared to MRSA strains [31].
In this study, the exfoliative toxin genes were found at a low frequency where the etb gene was detected in five strains (5.1%), four of them were MRSA while eta gene was detected in only one MRSA strain. Reports from studies carried out in Colombia [30] and Iran [32] showed similar results for eta gene prevalence. Low etb gene frequency was also reported in studies conducted in Iran [32], Nigeria [9], and the Czech Republic [31].
In the current study, the occurrence rate of exfoliative toxin genes in MRSA strains is higher than that in MSSA strains. This result is in agreement with the result of Liu’s group [33]. However, statistical analysis proved no significant difference in the distribution of these toxin genes between MRSA and MSSA strains which coincides with previously published data by Bukowski et al. [34].
Active efflux mediated by the two plasmid genes tetK and tetL and ribosomal protection encoded by tetM and tetO genes are the two main mechanisms for tetracycline resistance in S.aureus strains with a higher predominance of tetK and tetM genes [35]. In this study, tetK and tetM genes were harbored by 50.5% and 54.5% of the tested strains, respectively. In addition, both genes coexisted in 39% of strains. The aacA-aphD gene was detected in 32.3% of tested strains. Although a high percentage of MRSA group harbored aacA-aphD gene (39%) in comparison to MSSA group (20%), the difference is still statistically non-significant. The selective pressure encountered upon excessive use of aminoglycosides in the treatment of S.aureus infections may account for the increased prevalence rate of aacA-aphD gene among S.aureus strains. This selective pressure mediates the transfer and dissemination of aacA-aphD transposable resistance gene, and thus allows resistant clones to survive and circulate for long periods within health care facilities [36]. Similar results were reported by Khoramrooz et al. where resistance to tetracycline and aminoglycoside antibiotics was prominent in 47.93% and 30% of strains, respectively. However, they found a significant association between methicillin resistance and aminoglycoside resistance in tested strains [37]. In contrast, another study reported a low prevalence rate of tetk (6.1%), tetM (6.5%) and aacA-aphD (6.5%) genes with significant association of both tetK and tetM genes with mecA gene [38]. Three different mechanisms are involved in the resistance of S.aureus to macrolides, lincosamides, and streptogramin B of which ribosomal site methylation (mediated by erm genes where ermA and ermC are more common) and energy-dependent drug efflux (mediated by msrA gene) are well known. In the current study, msrA, ermA, and ermC genes were amplified in 44.4%, 17.2%, and 41.4% of S.aureus strains, respectively and ermA gene was found to have a significant association with mecA gene (P=0.025). In agreement with this data, Saderi et al. reported the distribution of ermC and ermA genes in 41.1% and 17.7% of tested strains, respectively [39]. A lower incidence rate of the three genes was recorded by Akpaka et al. where msrA, ermA, and ermC were found in 7.1%, 7.4%, and 0.6% of tested strains but same as this study, ermA gene was significantly associated with mecA gene-positive strains [38].
Based on Spearman’s rank correlation coefficient test, a significant positive correlation was found between lukD, lukE, and lukF genes. This may be attributed to the high predominance of the three genes in more than 50% of tested isolates. Also, positive significant correlation was detected between the following gene pairs; lukD/tetK, lukD/tetM, lukD/aacA-aphD, lukF/tetK, tetK/tetM, and msrA/aacA-aphD.
Analysis of toxin and resistance genes profiles reveals that 94.9% and 95.6% of isolates have at least one resistance gene and one toxin gene, respectively. MRSA strains were classified into 34 genotypes while MSSA strains had 17 genotypes with respect to resistance, which indicates the wide diversity of the resistance genes in MRSA strains.
CONCLUSION
The current study demonstrated a high prevalence of methicillin resistance among S.aureus clinical isolates in Egypt. The discrepancies found between phenotypic and genotypic methods for detection of methicillin resistance confirm that the presence of mecA gene is still the most accurate way to detect methicillin resistance in S.aureus strains. Analyzing data concerning the prevalence of some selected toxin and resistance genes showed that the highest prevalent toxin gene was lukE (93%) and the least prevalent one was eta (1%). In addition, tetM and tetK genes were amplified in nearly half of the tested S.aureus strains. Statistical analysis of data revealed no significant association between any of the detected genes and mecA gene except for ermA gene p<0.05. Noteworthy, the prevalence rate of methicillin resistance, leukocidin, exfoliative toxin genes, and the selected resistance genes differed from what was reported in other studies conducted in different countries and even those conducted in Egypt. This may be attributed to the variation in population awareness in the studied areas, the type of collected clinical specimens, infection control strategies, and treatment policies employed in hospitals where test specimens were collected.
ETHICS APPROVAL AND CONSENT TO PARTICIPATE
The present study was authorized by the research ethics committee, faculty of pharmacy, Mansoura University, Egypt (Code 2021 - 237).
HUMAN AND ANIMAL RIGHTS
No animals were used in this research. All human research procedures followed were in accordance with the ethical standards of the committee responsible for human experimentation (institutional and national) and with the Helsinki Declaration of 1975, as revised in 2013.
CONSENT FOR PUBLICATION
Informed consent was obtained from the participants.
STANDARDS OF REPORTING
STROBE guidelines were followed.
AVAILABILITY OF DATA AND MATERIALS
The data that supported the findings in this study are available upon request from the author [R.M.E].
FUNDING
None.
CONFLICT OF INTEREST
The author declares no conflict of interest, financial or otherwise.
ACKNOWLEDGEMENTS
The author expresses her deep thanks to Dr. Hanan Shehata, University of Guelph, Ontario, Canada for editing and reviewing English language in this manuscript.