All published articles of this journal are available on ScienceDirect.
Plant Growth-promoting and Bio-control Activity of Micrococcus luteus Strain AKAD 3-5 Isolated from the Soybean (Glycine max (L.) Merr.) Rhizosphere
Abstract
Background:
Applications of bioinoculants for improving crop productivity may be an eco-friendly alternative to chemical fertilizers. Rhizosphere or soil-inhabiting beneficial microbes can enhance plant growth and productivity through direct and indirect mechanisms, i.e., phosphate solubilization, nutrient acquisition, phytohormone production, etc.
Objective:
This study is based on the hypothesis that diseases resistant plants can act as a source of potential microbes that can have good plant growth-promoting traits and bio-control potential.
Methods:
In this study, we have isolated the rhizobacterial strains (AKAD 2-1, AKAD 2-10, AKAD 3-5, AKAD 3-9) from the rhizosphere of a disease-resistant variety of soybean (JS-20-34) (Glycine max (L.) Merr.). These bacterial strains were further screened for various plant growth-promoting traits (phosphate solubilization, indole acetic acid (IAA), ammonia, biofilm, HCN, Exopolysaccharide (EPS), and enzyme production activity (catalase, cellulase, and chitinase)).
Results:
Among four, only bacterial strain AKAD 3-5 has shown plant-growth-promoting and biocontrol (98%) activity against Fusarium oxysporum. Morphological, biochemical, and molecular characterization (16S rRNA) revealed that this rhizobacterial isolate AKAD 3-5 closely resembles Micrococcus luteus (Gene bank accession: MH304279).
Conclusion:
Here, we conclude that this strain can be utilized to promote soybean growth under varied soil stress conditions.
1. INTRODUCTION
Soybean has turned out to be a major oilseed crop in India, and a good source of protein supplement, wherein more than 40% population suffers from energy-protein malnutrition [1, 2]. By the year 2050, global food production needs to be doubled to feed 9.1 billion people of the world, as reported by FAO [3]. To achieve this ambitious goal, cereal production must be increased, with a high emphasis on sustainability. Therefore, the development of eco-friendly strategies can help to ameliorate the effect of abiotic and biotic stress in plants, and there is an urgent need for such strategies in our agricultural systems that have to cope with the impact of climate change [4-7]. The beneficial role of plant growth-promoting rhizobacteria (PGPR) and arbuscular mycorrhizal fungi (AMF) in improving plant growth under stressful conditions are well documented in the literature [1, 8-12]. Various reports are available demonstrating the role of PGPRs in mitigating abiotic stress in many crops, such as tomato, pepper, pea, maize, wheat, mung bean, and chickpea [13-19]. PGP microbes-induced plant growth potential is explained through numerous anticipated mechanisms [9, 20-22]. Several plant growth-promoting (PGP) microbes help to increase the availability of nutrients, like nitrogen (N), phosphorus (P), iron (Fe), as well as produce metabolites that can regulate growth and defense responses in plants [10, 23]. These microbes also produce plant hormones like auxin, cytokinin, gibberellic acid, abscisic acid, strigolactones, brassinosteroids, salicylic acid, jasmonic acid, and ethylene [24-26]. Groups of some plant growth-promoting (PGP) microbes produce some defensive molecules, like melatonin, proline, and carotenoids which play a defensive role under abiotic stress conditions. The study conducted by Zahedi and Abbasi [27, 28] investigated the effect of inoculation of three beneficial bacterial strains Azotobacter chroococcum, Azospirillum brasilense, and Rhizobium japonicum, and their combination on polyamines and phytohormones of soybean growing under different irrigation systems. The study conducted by Martins et al. [28] used three bacterial strains, Bacillus cereus, B. subtilis, and B. thuringiensis, for mitigating the effect of drought stress in soybean. Previous studies conducted on Micrococcus luteus were majorly focused on exploring its plant growth-promoting attributes [29-32], but plants under stress conditions are susceptible to different kinds of disease. In nature, plants are concurrently exposed to a combination of abiotic and biotic stresses that limits crop production [33]; however, the study related to PGPRs combating both abiotic and biotic stress is often limited. In the present study, we have isolated, identified, and screened a potential isolate Micrococcus luteus, from a disease-resistant variety of soybean (JS-20-34), with their effective plant growth-promoting, desiccation-tolerant, antifungal, and antagonistic activity against Fusarium oxysporum. The experimental work performed is shown in Fig. (1).
2. MATERIALS AND METHODS
2.1. Soil Sampling
Rhizosphere soil samples from a disease-resistant variety of soybean (JS-20-34) were collected by grubbing up soybean plants and the roots with attached soils from Jawaharlal Lal Nehru Krishi Anusandhan Kendra (JNKV) Sagar M.P. The location of the sampling site is at 23°49’44 N latitude and 78°42’46 E longitude. These rhizosphere soil samples were placed in sterile polythene bags, labelled, and stored at 4ºC in a refrigerator until use.
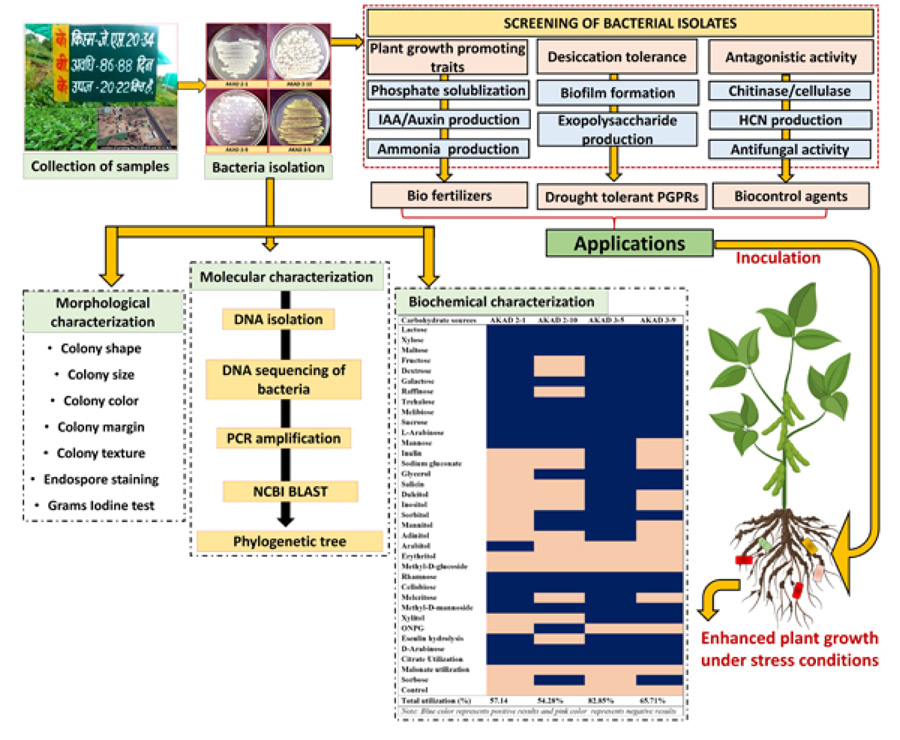
2.2. Isolation of Rhizobacteria:
Aliquots of 1 g rhizosphere soil were diluted with 9 mL of a sterile 0.9% NaCl solution. Serial dilutions were carried out and streaked onto Nutrient Agar (NA) plates (1 M NaOH, NaCl 5.0g/L, peptone 10g/L, 10ml/L, agar 15g/L, yeast extract 10g/L, and pH was maintained at 7) medium and the plates were incubated at 30 °C for 24 h and stored in glycerol at 4 °C. Rhizobacterial strains were cultured in the nutrient broth media and maintained in glycerol at -20°C.
2.3. Morphological and Biochemical Characterization
Bacterial isolates were morphologically identified based on the shape, size, color, and texture of colonies. The Grams staining was performed by following the protocol of Vincent and Humphrey [23]. Carbohydrate and the biochemical test were performed by using (KB009 HiCarbohydrateTM Test kit and KB002 HiAssortedTM Biochemical Test Kit). The kit was opened aseptically, and 50μl of pure bacterial cultures were inoculated to each well by using the surface inoculation method and incubated at 37°C for 1 day [11]. These biochemical tests are based on the principle of color change, change in pH, and substrate utilization by bacteria isolates.
2.4. Molecular Identification
Bacteria genomic DNA was extracted by using Bacterial g DNA Mini Kit according to the manufacturer's protocol. The quality and purity of isolated DNA were checked at 260/280 nm absorbance using a spectrophotometer. PCR amplification of the 16S rRNA gene was done by using universal primers 27F (AGAGTTTGATCMTGGCTCAG) and 1492R (TACGGTACCTTGTTACGACTT) and amplified by PCR using an automated thermal cycler (Biorad, Hercules, USA) by the following steps: denaturation at 94°C for 5 minutes followed by 30 cycles of amplification for 30 seconds at 94°C, annealing for 60 seconds at 52°C, extension for 2 minutes at 72°C, and elongation for 10 minutes at 72°C. Amplified products were visualized using 1% agarose gel and were sent for sequencing (outsourced). Obtained 16S rRNA gene sequences were blast on the NCBI BLAST database (http://www.ncbi.n1m.nih.gov/blast/Blast.cgi) and were compared to other sequences already present in the Gene Bank. These bacterial sequences were deposited to NCBI GenBank for accession numbers. The phylogenetic analysis of sequences with the closely related sequence of NCBI blast results was performed by using the automated NCBI pipeline. A phylogenetic tree of AKAD 3-5 was constructed by using iTOL (https://itol.embl.de/) after establishing a relationship with already available bacterial sequences [34].
2.5. Screening for PGP Traits
2.5.1. Phosphate Solubilizing Activity
The qualitative phosphate solubilization potential of bacteria was estimated by observing the large clear/halo zones on Pikovskaya agar media [35]. Phosphate solubilization activity was performed by growing the bacterial culture on Pikovskaya’s agar plate and then incubating the plate at 37ºC for 3 days following protocols [36]. The development of the clear halo zone around the bacterial colony specifies the positive result for phosphate solubilization. For quantitative estimation of phosphate solubilization, 1 ml of bacterial cell suspension (3 ×107 cells/ml) was inoculated in 50 ml of Pikovskaya's liquid medium (pH 7) in 150 ml Erlenmeyer flasks and incubated for a week in an orbital shaking incubator (Remi). It was followed by centrifugation of bacterial cell suspension for 10 min at 10,000 rpm. The phosphate content in the supernatant was estimated spectrophotometrically by the ascorbic acid method. The pH of the medium was recorded with a digital pH meter [37]. Soluble P (µg/mL) was estimated by plotting standard curve of KH2PO4.
2.5.2. Ammonia Production
Bacteria were tested for the production of ammonia in the liquid media containing peptone water and NaCl. 200µl of fresh bacterial culture was inoculated in 20 ml peptone broth and incubated for about 2-3 days at 37°C. Change in color from yellow to orange after the addition of Nessler's reagent was considered as a positive test for the production of ammonia [38].
2.5.3. Production of Indole-3-Acetic Acid
Bacterial cultures (100 µl) were added to Dev tryptophan broth (HiMedia) and allowed to grow by incubating at 37°C for 5 days on a rotary shaker [39]. The bacterial culture was then centrifuged for 20 min at 1000 rpm. Then 2 ml of Salkowsky’s reagent (containing 2 mL 0.5MFeCl3 and 48 mL 35% HClO4) was added to 1 ml of cell-free supernatant. Therefore, the development of the pink color was considered as a positive result for IAA/auxin production. IAA production was measured by recording the absorbance at 530 nm using a UV-Vis spectrophotometer. A standard curve of commercial Auxin/IAA was plotted for quantification of auxin in the solution, and an un-inoculated medium served as a control. The amount of IAA in the culture was expressed as µg/ml.
2.5.4. Biofilm Formation
Biofilm forming ability of bacterial strains was assayed using CV (crystal violet) by following the methods of Feoktistova et al. [33]. Bacterial strains were grown in nutrient broth for about 3-4 days and incubated at 37°C. After incubation, bacterial cell cultures were stained by using a crystal violet solution (1%) and extracted with ethanol. The biofilm formation by bacterial strains was quantified by recording the absorbance at 570 nm in a 96 well microliter plate reader.
2.5.5. Exopolysaccharide Production (EPS)
For exopolysaccharide production (EPS), Micrococcus luteus cells were grown in EPS media by Kimmel et al. [39]. Bacterial strain AKAD 3-5 was grown at 37 °C for 72 hrs with constant shaking at 150 rpm. Cell-free extracts were collected by centrifugation 25 °C at 16000 rpm for 15 min and filtered through a 0.2 µm filter. Exopolysaccharides were precipitated by using cold acetone and kept at -20°C for 2-3 hrs. The precipitate was separated by centrifugation at 16000 rpm for 15 min at 4°C and re-suspended in distilled water. This suspension was lyophilized and stored at -20°C. EPS concentration was determined by the phenol sulfuric acid method, described previously by Dubois et al. [40]. The concentration of EPS was determined with reference to a standard curve prepared with glucose.
2.5.6. Screening of Isolates for HCN
Hydrogen cyanide (HCN) production by bacterial strains was tested by using the method of Lorck [41]. Bacterial colonies were streaked on a nutrient agar plate supplemented with 4.4 g/l of glycine. Therefore, the production of cyanide was detected by placing Whatman filter paper No.1 saturated in a 0.5% picric acid solution. The change in yellow to red color of filter paper after 48 hrs of incubation specified positive results for HCN production.
2.5.7. Assessment of Bacterial Isolates for Enzymatic Activity
For catalase activity, 100µl of 3% hydrogen peroxide was added to 24 hrs old bacterial culture in Eppendorf tubes. The instant bubble formation is considered a positive test for catalase activity [42]. Cellulase production was determined by following the methods of Kasana et al. [43]. Bacterial strains were inoculated into the plates containing the media (CMC, NaNO3, KCl, K2HPO4, MgSO4, peptone, yeast extract, and agar) and incubated for 3-5 days at 35 °C. Then these CMC agar plates were flooded with grams iodine solution and allowed to stand for about 1 min at room temperature, and 1M NaCl solution was used for counterstaining. Clear halos zones were observed around bacterial colonies indicating hydrolysis of cellulose [43]. Chitinase activity was measured by following the protocol of Babashpour et al. [44]; therefore, the development of clear halos zones was observed around growing bacterial colonies, indicating hydrolysis of chitin (Table 1).
2.5.8. Bio-control Activity against Fusarium oxysporum
For testing the bio-control potential of different bacterial isolates against F. oxysporum, selected bacterial isolates were co-cultured by using a dual plate culture assay. A fungal disc (2 mm) was placed in the center, and bacterial isolates were inoculated at four opposing corners on the PDA plates. The cultures were incubated at 28°C for 4-5 days, after which the diameter of the fungal and bacterial colony was measured. Antifungal activity was expressed as a percentage (%) inhibition rate calculated by the formula C-T/C×100, where C and T are the fungal mycelial diameter on control and the test plate, respectively [45, 46].
3. RESULTS AND DISCUSSION
In the present study, four rhizobacterial isolates were successfully isolated from the rhizosphere of a disease-resistant variety of soybean (JS-20-34). These four bacterial isolates (AKAD 2-1, AKAD 2-10, AKAD 3-5, AKAD 3-9) were identified morphologically based on color, shape, size, margin, the texture of colonies, and grams staining. Most of the bacterial strains were rod shape, gram-negative, and forming a white color colony except AKAD 3-5 strain. Bacterial isolate AKAD 3-5 were found to be gram-positive, non-motile, coccus shape, forming smooth yellow colonies (Table 2).
Bacterial isolates were tested for their carbohydrates utilization potential against 35 different carbohydrate sources. Bacterial isolate AKAD 3-5 had utilized maximum (82.85%) carbohydrate sources, followed by AKAD 3-9, which utilized 65.71% carbohydrate sources, as shown in Table 1. Bacterial strains AKAD 2-1 and AKAD 2-10 utilized only 57.14% and 54.28% of carbohydrate sources, respectively. AKAD 3-5 was further used for biochemical characterization, and it utilized almost all the (91.6%) biochemical sources like ornithine, urease, adonitol, phenylalanine deamination, lysine utilization, nitrate reductase, arabinose, glucose, lactose sorbitol citrate utilization, except H2S. Strain AKAD 3-5 was identified as Micrococcus luteus (Gene bank Accession no MH304279), showing 98% similarity and 100% query cover with Micrococcus luteus strains. The phylogenetic tree was prepared by using the iTOL v3 (Interactive tree of life) (Fig. 2). This strain has the potential to promote plant growth, as shown by its various activities that can be used for promoting soybean and other plant growth under soil stress conditions.
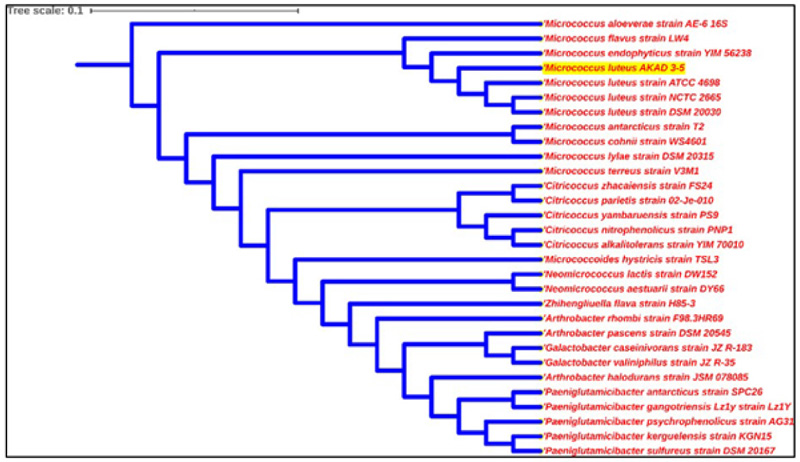
These rhizobacterial isolates were further screened for various plant growth-promoting traits (phosphate solubilization, indole acetic acid (IAA), ammonia production, biofilm production, HCN production, catalase, chitinase, and cellulase production). All the bacterial isolates were selected as phosphate solubilizers and produced a clear halo zone ranging from 10-13 mm. Micrococcus luteus AKAD 3-5 strain was found to be the best phosphate solubilizer by producing 135 μg mL-1 of phosphate. Our study was supported by the study conducted by Raza and Faisal [32], who reported that phosphate solubilizing bacteria Micrococcus luteus-chp37 can tolerate desiccation and enhance plant growth in maize by producing plant growth-promoting compounds hydrogen cyanide (HCN) and cytokine (Fig. 2). Phosphate solubilizing bacteria (PSB) have been shown to solubilize and mineralize phosphorous from inorganic and organic sources of total soil P, thereby increasing the availability of the P to the plants, which may promote plant growth [47]. Three bacterial isolates (AKAD 2-1, AKAD 2-10, and AKAD 3-5) were forming biofilm, and bacterial strains AKAD 3-5 possesses a strong biofilm-forming capacity (2.05±0.1). It was validated previously that biofilm-producing bacteria play an imperative role in improving soil fertility and bioremediation [48]. Our study reported that 440 µg mL-1. EPS production was recorded in Micrococcus luteus when cells were grown in EPS medium (Table 3). Most of the functions attributed to EPSs are defensive. However, some studies describe some direct evidence illustrating the role of biofilm formation and exopolysaccharides production in protection against abiotic and biotic stresses, especially drought stress [47-49].
All the bacterial isolates showed a positive result for ammonia production (Fig. 3) in the range of 2.45-5.15 μg mL-1 and AKAD 3-5 produced 34.4 μg mL-1 of IAA (Table 3). Therefore, it was well reported that IAA-producing microbes help in enhancing the surface area, root length, and also relax plant cell walls, which enables better nutrient uptake and creates strong plant-microbe communication by amassing more root exudates [50]. Production of HCN, hydrolytic, or cell wall degrading enzymes by bacterial isolate AKAD 3-5 enzymes may be attributed to being used as biocontrol agents against pathogen attack. The production of extracellular enzymes such as cellulase, chitinase, and protease can hydrolyze cell wall components consisting of proteins and chitin, β- 1, 3- glucanase. They have been shown to mediate the bio-control of fungal pathogens [43]. Enzymes like cellulases produced by beneficial bacteria play a very important role in bacterial penetration inside the plant and help in plant colonization [51, 52] (Fig. 3).
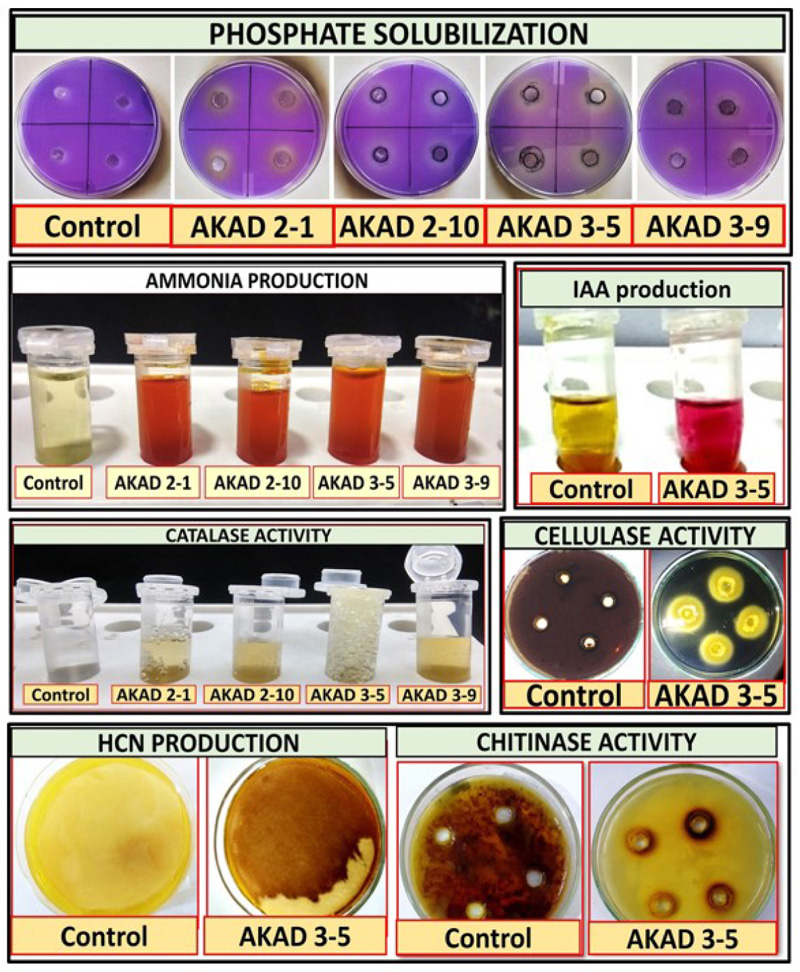
![]() |
After testing for various cell wall degrading enzymes (chitinase and cellulase) and HCN production, these bacterial strains were further tested for their inhibitory effect on Fusarium oxysporum (ITCC 2389). Only Micrococcus luteus strain AKAD 3-5 showed 98% inhibition against mycelia growth of Fusarium oxysporum (ITCC 2389) in dual plate culture assay (Fig. 4). Similar observations were made by Patel et al. [52], reported plant-growth-promoting and biocontrol activity of marine bacteria M. luteus and tested their effects on vegetative parameters. The results from this study suggested that M. luteus can protect chickpea from Fusarium wilt disease as it displayed strong biocontrol ability against fungal pathogens and acted as a promising plant growth-promoting bacterial strain to enhance overall plant growth in chickpea [51]. Most of the studies conducted by different scientists have shown the positive plant-growth-promoting [30, 52-54], desiccation-tolerant [32], and biocontrol properties [53] of M. luteus individually, but the present study showed plant-growth-promoting together with abiotic and biotic stress tolerant potential of M. luteus AKAD 3-5 strain compared to other reported studies (Table 4).
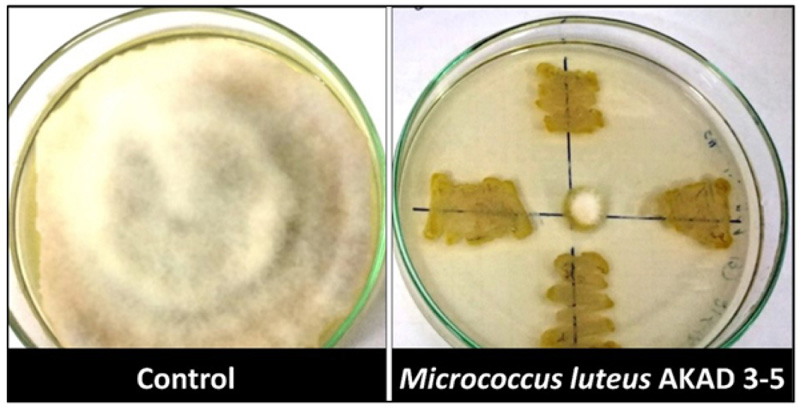
Morphological Characteristics |
Micrococcus luteus AKAD 3-5 strain |
Biochemical Utilization | Micrococcus luteus AKAD 3-5 Strain |
---|---|---|---|
Colony shape | Circular | Citrate Utilization | Negative |
Colony color | Yellow | Lysine Utilization | Positive |
Colony elevation/ margin | Convex /Entire | Ornithine Utilization | Positive |
Cell shape | Cocci | Urease | Positive |
Gram staining | Gram-positive | Phenylalanine Deamination | Positive |
Capsules staining | Positive | Nitrate reduction | Positive |
Spore staining | Positive | H2S Production | Negative |
Motility | Non-motile | Glucose | Positive |
Isolate |
IAA (μg mL-1) |
Ammonia (μg mL-1) |
Phosphate Solubilization (μg mL-1) |
Biofilm (OD at 570 nm) |
EPS Production (µg mL-1) |
---|---|---|---|---|---|
AKAD 2-1 | - | 3.10±0.02 | 50.3 ± 1.34 | - | - |
AKAD 2-10 | - | 5.15±0.09 | 110.2 ± 31 | - | - |
AKAD 3-5 | 34.4 ± 0.23 | 4.6±0.03 | 135 ± 2.4 | 2.05 ± 0.1 | 440 ± 3.56 |
AKAD 3-9 | - | 2.45±0.021 | 90.45 ± 2.45 | - | - |
Note: Data presented is the mean of three replicate ± SD (n=3) |
Bacterial Strain | IAA | Ammonia |
Phosphate Solublization |
Siderophore Production | Biofilm Activity | EPS Production | HCN Production | Chitinase | Cellulase | Antifungal Activity | References |
---|---|---|---|---|---|---|---|---|---|---|---|
Micrococcus luteus strain AKAD 3-5 | Present | Present | Present | NT | Present | Present | Present | Present | Present | Present | Present study |
Marine Micrococcus luteus strain | Present | Present | Present | Present | NT | NT | NT | Present | NT | Present | [53] |
Micrococcus luteus-chp37 | NT | NT | Absent | Absent | NT | Present | Present | NT | NT | NT | [32] |
Micrococcus sp NII-0909 | Present | NT | Present | Present | NT | NT | NT | NT | NT | NT | [54] |
Micrococcus luteus RWL-3 | Present | NT | NT | NT | NT | NT | NT | NT | NT | NT | [30] |
Micrococcus luteus strain 4.43 | Present | NT | NT | NT | NT | Present | NT | NT | NT | NT | [31] |
NT: not tested |
CONCLUSION
Different studies conducted on members belonging to Micrococcus sp. have proven their role as excellent biofertilizers due to their multifarious plant growth-promoting activity. However, the dual potential of these bacterial isolates is less explored. In the present study, we have isolated Micrococcus luteus from a disease-resistant variety of soybean (JS-20-34) with multifarious plant growth-promoting traits (Auxin/IAA, ammonia production, phosphate solubilization), desiccation-tolerant potential (biofilm and EPS production), colonization potential (cellulase activity), antifungal activity (chitinase, HCN production), and antagonistic activity against Fusarium oxysporum. Screening of these rhizobacteria from the rhizosphere of disease-resistant varieties of a plant may be a sustainable option to improve soil health and biomass production on marginal land. This study suggests that bacterial Micrococcus luteus strain AKAD 3-5 can be formulated into tailor-made bio-fertilizer and bio-control agent for promoting plant growth under abiotic and biotic stress conditions in soybean. The application of PGPR as an alternative to chemical fertilizers offers a sustainable, safe, and eco-friendly approach to maintain soil health and increase crop production.
ETHICS APPROVAL AND CONSENT TO PARTICIPATE
Not applicable.
HUMAN AND ANIMAL RIGHTS
Not applicable.
CONSENT FOR PUBLICATION
Not applicable.
AVAILABILITY OF DATA AND MATERIALS
The data supporting findings of this study is present within the article.
FUNDING
The authors are grateful to DST Inspire Ph.D. Fellowship (IF160797), DST Govt. New Delhi, India for funding this study.
CONFLICT OF INTEREST
The authors declare no conflict of interest, financial or otherwise.
ACKNOWLEDGEMENTS
Anamika Dubey would like to acknowledge her thesis supervisor, Ashwani Kumar, for the supervision and successful conduction of the work, manuscript writing, editing, and critical comments.