All published articles of this journal are available on ScienceDirect.
Diversity, Antimicrobial Susceptibility patterns, and Biofilm Formation of Staphylococcus spp. in Cosmetic Products in Western Saudi Arabia
Abstract
Background
Cosmetics have become essential for skincare, makeup, and hair care. Cosmetic products can be contaminated during production and application. This study investigated the staphylococci contamination parameters (virulence factors, diversity, and antimicrobial susceptibility patterns) in cosmetic products in Western Saudi Arabia.
Materials and Methods
A total of 250 cosmetic products were purchased from local outlets. Staphylococci prevalence was tested through standard microbiological culturing methods, whereas the Vitek-2 compact system confirmed the presence of different staphylococci genera and revealed its antimicrobial susceptibility patterns. Moreover, PCR protocols were performed to detect virulence factors encoding genes.
Results
The data revealed a low prevalence of Staphylococcus spp. in cosmetics (10.4%, n = 26). Lipstick, face powder, and blusher samples comparatively presented higher contamination rates. Staphylococcus xyloses, S. epidermidis, and S. aureus were among the identified species. The results of antimicrobial susceptibility patterns demonstrated benzylpenicillin resistance in most of the isolates (61.53%), whereas oxacillin and erythromycin resistance was noted in 26.9% of isolates. Contrarily, the isolates were not resistant to tigecycline, gentamicin, nitrofurantoin, or linezolid. Approximately 19.2% of the isolates exhibited resistance to multiple antimicrobial classes, indicating the presence of multidrug-resistant (MDR) strains. Methicillin-resistant Staphylococcus aureus (MRSA) was isolated from lipstick and concealer. Biofilm formation-encoding genes (icaA and icaB) were detected in 50% (4) of the potentially pathogenic isolates, whereas icaR, icaC, and icaD genes were noted in 37.5% (3) of the isolates. The mecA resistance gene was detected in 37.5% (3, n = 8) of Staphylococcus spp. isolates..
Conclusion
Poor quality cosmetic products may act as a medium for the transmission of potentially pathogenic, antibiotic-resistant Staphylococcus spp. The results necessitate proper storage and handling of cosmetic products to avoid microbial contaminations.
1. INTRODUCTION
Millions of global consumers daily apply cosmetics to improve their appearance. Cosmetic products do not require a prescription like medicines. Therefore, cosmetics-related regulations are comparatively less strict than prescription drugs [1, 2]. The cosmetic products’ formulations with a higher ratio of water and surfactants are more susceptible to microbial contamination. Minerals, plants, animal-based raw materials, and water content facilitate bacterial contamination in cosmetic products. Additionally, improper and lack of hygiene manufacturing practices could aid microbial contamination of cosmetics [3]. The opportunistic/pathogenic bacterial contamination in cosmetic products can transmit infections among consumers. The production environment and organic ingredients (lipids, sugar, organic acids, and proteins) also favor microbial contamination in unused cosmetic products [4]. The rising interest in skin microbiota has raised the attention of the cosmetic industry to maintain good health of the cutaneous barrier [5]. Microorganisms are crucial for maintaining the immune system and skin barrier, and restricting pathogenic growth [6]. Skin microbiota imbalance (dysbiosis) can cause various skin illnesses, such as dry and sensitive skin, atopic dermatitis, and acne [5]. Improper cosmetics handling can result in transmittable eye and skin infections [7, 8]. Skin products (cream and powder), eye products (eyeliner and mascara), and hairdressing procedures pose skin infection risks [9, 10].
Fungal pathogens such as Penicillium spp., and opportunistic yeasts such as Candida spp. and Rhodotorula spp., as well as bacterial pathogens including Pseudomonas aeruginosa and S. aureus, have been reported in beauty products [7-11]. Staphylococcus aureus is particularly significant regarding abscesses and skin infections such as scalded skin syndrome in humans [12, 13]. S. epidermidis is also known to cause skin infections, bullous impetigo, hair follicle infections, and boils, particularly in beauty and hairdressing salons Therefore, hygienic handling can restrict bacterial contamination and transmission among users of cosmetic products. S. epidermidis and S. aureus are sources of multiple hospital- and community-acquired human illnesses that have been reported in various studies [10, 11, 14].
Staphylococcus spp.-associated infections may not all be reported, however, the number of reported cases of S. aureus infections is increasing dramatically around the world [12]. It was estimated that more than 80000 cases of methicillin-resistant Staphylococcus aureus (MRSA)-associated infections reported in the United States alone in 2011, resulting in more than 10000 deaths [12], this figure may have been sharply increased in the past 10 years. The increased number of infections due to Staphylococcus spp., particularly S. aureus may be linked with multidrug-resistance patterns observed in Staphylococcus spp. In Saudi Arabia, Antibiotic resistant Staphylococcus spp. was detected in environmental and clinical settings [15-17]. A high prevalence of multidrug-resistant (MDR) S. aureus and S. haemolyticus was reported in Saudi Arabia, highlighting the significance of MDR Staphylococcus spp. in human infections [16, 17].
This study analyzed high and low-quality cosmetic products for Staphylococcus spp. prevalence and diversity. Biofilm forming capability and antimicrobial susceptibility profiles of detected Staphylococcus spp. were investigated as well. The study also explored antibiotic-resistant Staphylococcus spp. contamination in cosmetics, highlighting the associated health risks.
2. MATERIALS AND METHODS
2.1. Sampling
Factory-sealed and new samples (250) of different cosmetics were either purchased from malls and high street shops (branded high-quality products) or traditional markets (unbranded low-quality/counterfeit products) in Makkah, Saudi Arabia, from July to December 2022 (Table 1). The examination involved the assessment of external appearance, production and expiry dates, and manufacturing country.
Total Number of Samples | Branded | Non Branded | Code | Contaminated Products | No |
---|---|---|---|---|---|
51 | 3 | 48 | LP | Lipstick | 1 |
22 | 3 | 19 | PW | Face powder | 2 |
10 | 3 | 7 | BL | Blusher | 3 |
4 | 3 | 1 | SG | Shower gel | 4 |
29 | 3 | 26 | M | Mascara | 5 |
16 | 3 | 13 | EY | Eyeliner | 6 |
13 | 3 | 10 | C | Concealer | 7 |
15 | 0 | 15 | F | Foundation | 8 |
18 | 0 | 18 | Gl | Glitter | 9 |
6 | 0 | 6 | Lg | Lip gloss | 10 |
8 | 0 | 8 | LN | Lenses | 11 |
3 | 0 | 3 | Pr | Primer | 12 |
4 | 0 | 4 | Hi | Highlighter | 13 |
4 | 3 | 1 | Tp | Toothpaste | 14 |
11 | 0 | 11 | Fc | Face cream | 15 |
7 | 0 | 7 | Sc | Scrub cream | 16 |
7 | 0 | 7 | Mw | Mouth wash | 17 |
7 | 0 | 7 | Sh | Shampoo | 18 |
6 | 0 | 6 | Cw | Cleansing water | 19 |
2 | 0 | 2 | Cr | Body lotion | 20 |
4 | 3 | 1 | MR | Makeup remover | 21 |
3 | 3 | 0 | Es | Eye shadow | 22 |
250 | 30 | 220 | All products |
Gene | Primer Sequence | Annealing Temperature | Amplicon Size (bp) |
---|---|---|---|
icaR-F | 5`- TAA TCC CGA ATT TTT GTG AA-3` | 52.0 °C | 469 |
icaR-R | 5`- AAC GCA ATA ACC TTA TTT TCC-3` | ||
icaA-F | 5`- ACA GTC GCT ACG AAA AGA AA- 3` | 53.2 °C | 103 |
icaA-R | 5 - GGA AAT GCC ATA ATG ACA AC- 3` | ||
icaD-F | 5 - ATG GTC AAG CCC AGA CAG AG- 3` | 53.5 °C | 198 |
icaD-R | 5` CGT GTT TTC AAC ATT TAA TGC AA-3` | ||
icaB -F | 5`- CTG ATC AAG AAT TTA AAT CAC AAA- 3` | 53.2 °C | 302 |
icaB -R | 5`- AAA GTC CCA TAA GCC TGT TT-3` | ||
icaC-F | 5`- TAA CTT TAG GCG CAT ATG TTT T-3` | 52.8 °C | 400 |
icaC- R | 5`- TTC CAG TTA GGC TGG TAT TG-3` | ||
mecA - F | 5`- AAAATCGATGGTAAAGGTTGGC- 3` | 55.0 °C | 533 |
mecA - R | 5`- AGTTCTGCAGTACCGGATTTTGC- 3` |
2.2. Detection and Identification of Staphylococcus spp.
The enrichment and selective plating were performed to detect Staphylococcus spp. in cosmetics. Briefly, powdered and solid products (1 g) were aseptically added to peptone water (9 mL) (Oxoid, UK) in screw-top universal bottles. The enrichment was carried out by aerobically incubating (37 °C) for 24-48 h. After incubation, the enrichment bottles with turbidity were considered positive for microbial contamination. Each enrichment sample was individually streaked on mannitol salt agar (MSA) (Oxoid) plates and aerobically incubated (37 °C) for 24-48 h [15]. MSA plates presenting yellow colonies with yellow surrounding zones (S. aureus colonies) and colorless or pink colonies with red surrounding zones (staphylococci colonies other than S. aureus) were considered presumptive positive results. The sheep blood (5%) supplemented Columbia blood agar (Oxoid) was used to purify presumptive Staphylococcus spp. colonies. Three colonies were selected from each plate for the purification process [16, 17]. Staphylococcus spp. presumptive colonies were further confirmed through a clumping factor test (MastStaph kit, Mast Diagnostics, UK), Gram staining, catalase test, hemolytic activity on sheep blood agar (Oxoid), and deoxyribonuclease production on DNAse agar (Oxoid) [15-17]. The Vitek® 2 compact system the (BioMérieux Inc., France) with GP and AST-N026 cards was used for further confirmation of Staphylococcus species and CoNS genera differentiation. The cards were inoculated according to the manufacturer’s guidelines and incubated in the system. The Advanced Expert System (AES, version 8.0) was employed to analyze the outputs [16, 17].
2.3. Antimicrobial Susceptibility Testing
Antimicrobial susceptibility patterns of confirmed Staphylococcus spp. were evaluated using the Vitek® 2 compact system (BioMérieux Inc.). The estimation of susceptibility patterns of 16 antibiotics from 13 antimicrobial classes was based on their minimum inhibitory concentrations (MIC). The antibiotics included penicillins [oxacillin and benzylpenicillin], lincosamides [clindamycin], macrolides [erythromycin], tetracyclines [tetracyclines], ansamycins [rifampicin], folate pathway anatgonists [tirmethoprime/sulfamethoxazole], nitrofurans [nitrofurantoin], fluoroquinolones [moxifloxacin, ciprofloxacin, and levofloxacin], aminoglycosides [gentamicin], glycopeptides [vancomycin], streptogramin [quinupristin], glycylcyclines [tigecycline], and oxazolidinones [linezolid] [16, 17].
Clinical and Laboratory Standards Institute [ 18 ] guidelines were followed to interpret Staphylococcus spp. antimicrobial susceptibility patterns. The Vitek 2 compact system was used for cefoxitin and inducible clindamycin resistance screening tests.
2.4. Detection of Biofilm Formation and Methicilin-resistance-encoding Genes
A standard PCR protocol was adopted to detect biofilm formation-encoding genes (icaR, icaA, icaD, icaB, icaC). Briefly, an overnight incubated bacterial culture on brain-heart infusion agar plates (Oxoid) was used for genomic DNA extraction. Bacterial colonies (1-2) were resuspended in sterile distilled water (20 mL) and incubated (100 ºC) for 20 minutes. A 5 μL aliquot of this suspension was used as the template DNA for PCR amplification in a thermal cycler (UNO II Thermocycler, Germany). Table 2 depicts the primer sequences (forward and reverse), and amplicon sizes of icaR, icaD, icaB, icaC, and icaA genes. The reaction mixture (25 μL) consisted of reverse and forward primers (2.5 μL of 1 M each), DNA template (5 μL of 150 ng), EzWay TMPCR Master Mix (10 μL) (LabisKoma, Korea), Taq DNA polymerase (1 U), and distilled water (5 μL). The amplification protocol included initial denaturation (94°C for 5 minutes) followed by 50 cycles of denaturation, annealing, and extension [(94°C for 30 s), (55.5°C for 30 s), (72°C for 30 s)], and a final extension (72°C for 1 minute). Furthermore, ethidium bromide-stained agarose gel electrophoresis (2% agarose in Tris-borate-EDTA) was performed using amplified PCR products (10 μL). The Gene Ruler DNA ladder (Koma Biotech Inc., Korea) of 100 bp served as the DNA marker [19]. The methicillin resistance (mecA) gene was also detected through the standard PCR method. Table 2 presents the primer sequences and relevant amplicon sizes. Biofilm formation-encoding genes were detected according to the same PCR protocol as mentioned above [20].
3. RESULTS
3.1. Prevalence and Diversity of Staphylococcus spp. in Cosmetic Products
The results demonstrated 26 (10.4%) Staphylococcus spp. positive samples out of 250 samples of cosmetic products (Table 3). Product type-based further classification revealed the highest contamination (50%) in Blusher samples followed by 25%, 18.9%, 17.6%, 13.8%, 12.5%, and 7.7% in shower gel, face powder, lipstick, mascara, eyeliner, and concealer, respectively (Table 3). S. xylosus had the highest prevalence of 23.08% among Staphylococcus spp. isolates followed by 19.23%, 15.38%, 11.54%, and 11.54% of S. epidermidis, S. warneri, S. hominis, and S. sciuri, respectively. S. lentus and S. aureus had a similar prevalence of 7.69%, whereas the lowest prevalence of S. haemolyticus was noted as 3.84% (Table 4).
Source of Isolates | No. of Examined Samples | Positive Samples | Negative Samples | ||
---|---|---|---|---|---|
No | % | No | % | ||
Lipstick (LP) | 51 | 9 | 17.6 | 42 | 82.35 |
Blusher (BL) | 10 | 5 | 50 | 5 | 50 |
Face powder (PW) | 22 | 4 | 18.18 | 18 | 81.81 |
Mascara(M) | 29 | 4 | 13.79 | 25 | 86.20 |
Eyeliner (EY) | 16 | 2 | 12.5 | 14 | 87.5 |
Concealer(C) | 13 | 1 | 7.69 | 12 | 92.30 |
Shower gel (SG) | 4 | 1 | 25 | 3 | 75 |
Glitter (GL) | 18 | 0 | 0 | 18 | 100 |
Lip gloss (LG) | 6 | 0 | 0 | 6 | 100 |
Lenses (LN) | 8 | 0 | 0 | 8 | 100 |
Foundation (F) | 15 | 0 | 0 | 15 | 100 |
Primer (Pr) | 3 | 0 | 0 | 3 | 100 |
Highlighter (Hi) | 4 | 0 | 0 | 4 | 100 |
Toothpaste (Tp) | 4 | 0 | 0 | 4 | 100 |
Face cream (Fc) | 11 | 0 | 0 | 11 | 100 |
Scrub cream (Sc) | 7 | 0 | 0 | 7 | 100 |
Mouthwash (Mw) | 7 | 0 | 0 | 7 | 100 |
Shampoo (Sh) | 7 | 0 | 0 | 7 | 100 |
Cleansing water (Cw) | 6 | 0 | 0 | 6 | 100 |
Body lotion (Cr) | 2 | 0 | 0 | 2 | 100 |
Makeup remover (MR) | 4 | 0 | 0 | 4 | 100 |
Eye shadow (es) | 3 | 0 | 0 | 3 | 100 |
All products | 250 | 26 | 10.4 | 224 | 89.6 |
P(a) | <0.001 | P(b) | <0.001 |
Species | Number of Isolates | % |
---|---|---|
S. aureus | 2 | 7.69 |
S. epidermidis | 5 | 19.23 |
S. lentus | 2 | 7.69 |
S. hominis ssp. hominis | 3 | 11.54 |
S. sciuri | 3 | 11.54 |
S. warneri | 4 | 15.38 |
S. xylosus | 6 | 23.08 |
S. haemolyticus | 1 | 3.84 |
P(a) | <0.001 | |
P(b) | 0.001> |
3.2. Antimicrobial Susceptibility Patterns of Staphylococcus spp. in Cosmetic Products
The data of antimicrobial susceptibility patterns of Staphylococcus spp. illustrated that 61.53% of Staphylococcus spp. isolates were resistant to benzylpenicillin (Penicillins), whereas resistance to tetracycline (Tetracyclines) was noted in 30.76% of isolates. The resistance to oxacillin (Penicillins) and erythromycin (Macrolides) was noted in 26.92% of isolates, whereas 19.23% of isolates were resistant to clindamycin (Lincosamides) (Table 5). A comparatively lower resistance of 7.69% and 3.84% was noted in trimethoprim/sulfamethoxazole (folate pathway antagonists), and rifampicin, respectively (Table 5). None of the Staphylococcus spp. isolates were resistant to all tested antibiotics (fluoroquinolones, streptogramin, glycylcyclines, aminoglycosides, oxazolidinones, nitrofurans, and glycopeptides) (Table 5). Five Staphylococcus spp. isolates, including four S. xylosus and one S. lentus isolate, exhibited multidrug resistance (MDR) (Table 6). S. epidermidis and S. aureus isolates did not exhibit MDR patterns (Table 6). Multidrug resistance patterns included resistance to rifampicin, benzylpenicillin, trimethoprim/sulfamethoxazole, oxacillin, clindamycin, erythromycin, and tetracycline (Table 6).
Antibiotics | Drug Class |
No. of Resistant (%) Staphylococcus spp. (n= 26) |
---|---|---|
Benzylpenicillin Oxacillin |
Penicillins | 16 (61.53) 7 (26.92) |
Erythromycin | Macrolides | 7 (26.92) |
Clindamycin | Lincosamides | 5 (19.23) |
Tetracycline | Tetracyclines | 8 (30.76) |
Trimethoprim/Sulfamethoxazole | Folate pathway antagonist |
2 (7.69) |
Rifampicin | Ansamycins | 1 (3.84) |
Ciprofloxacin Levofloxacin Moxifloxacin |
Fluoroquinolones | 0 (0) 0 (0) 0 (0) |
Gentamicin | Aminoglycosides | 0 (0) |
Nitrofurantoin | Miscellaneous | 0 (0) |
Vancomycin | Glycopeptides | 0 (0) |
Tigecycline | Glycylcyclines | 0 (0) |
Linezolid | Oxazolidinones | 0 (0) |
Quinupristin | Streptogramin | 0 (0) |
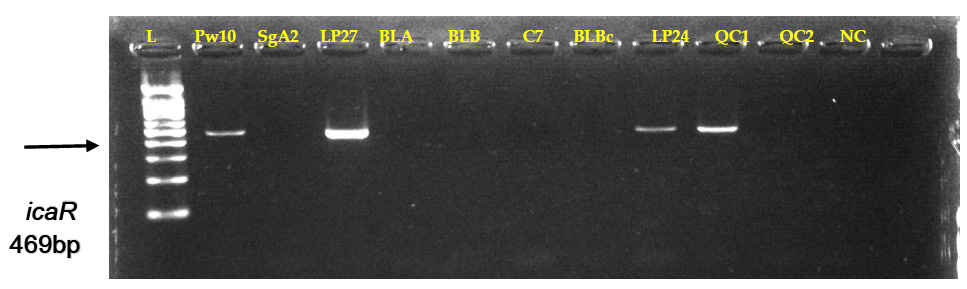
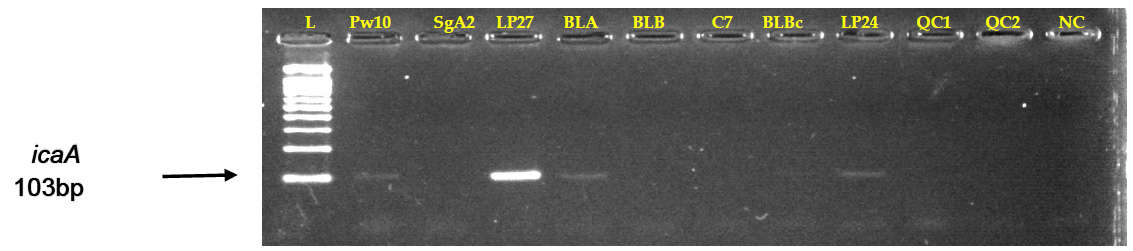
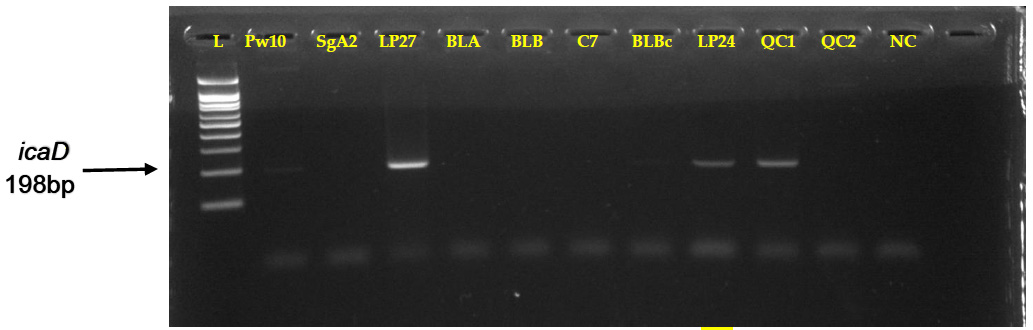
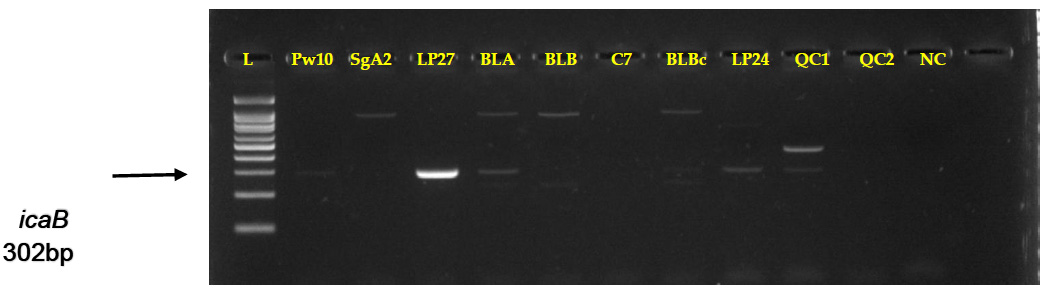
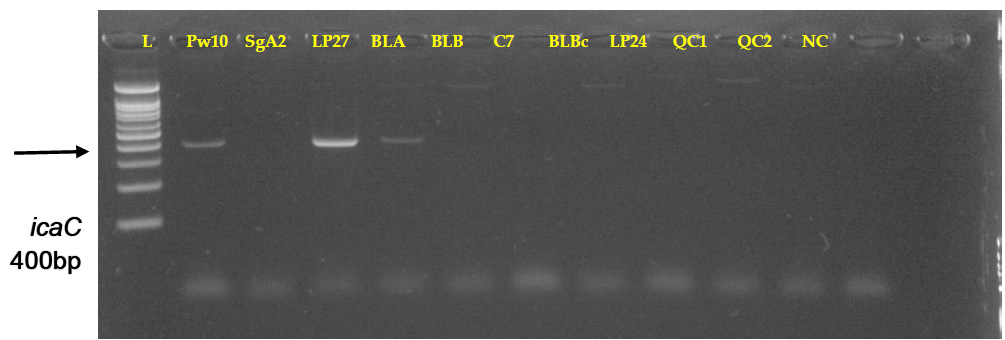
Isolate Code | Species | Origin | Resistance Pattern | No. of Classes | Resistance Pattern | Cefoxitin Screening* | Inducible Clindamycin Resistance* |
---|---|---|---|---|---|---|---|
LP3 | S. lentus | Lipstick | BEN/ OX | 1 | - | - | - |
LP24(1) | S. aureus | Lipstick | BEN/ OX/ TET | 2 | - | + | - |
LP27 | S. epidermidis | Lipstick | BEN/ E | 2 | - | - | - |
LP33A | S. hominis ssp. hominis | Lipstick | BEN/ OX/ TET | 2 | - | + | - |
LP33B | S. sciuri | Lipstick | 0 | 0 | - | - | - |
LP38A | S. xylosus | Lipstick | E/ CL/ TET/ TRIM | 4 | MDR | - | - |
LP38B | S. xylosus | Lipstick | BEN/ OX/E/ TET/ TRIM | 4 | MDR | + | - |
LP39A | S. xylosus | Lipstick | E/ CL/ TET | 3 | MDR | - | - |
LP39B | S. xylosus | Lipstick | E/ CL/ TET | 3 | MDR | - | - |
BL3 | S. sciuri | Blusher | 0 | - | - | - | |
BL5 | S. sciuri | Blusher | BEN | 1 | - | - | - |
BLA | S. epidermidis | Blusher | BEN | 1 | - | - | - |
BLB | S. epidermidis | Blusher | BEN | 1 | - | - | - |
BLBc | S. epidermidis | Blusher | BEN | 1 | - | - | - |
PW1 | S. xylosus | Face powder | 0 | 0 | - | - | - |
PW2 | S. warneri | Face powder | BEN | 1 | - | - | - |
PW10 | S. haemolyticus | Face powder | BEN/ OX | 1 | - | + | - |
PW14(2) | S. lentus | Face powder | BEN/OX/CL/ RIF | 3 | MDR | + | - |
M2A | S. warneri | Mascara | BEN/E | 2 | - | - | - |
M2A1 | S. hominis ssp. hominis | Mascara | BEN/E | 2 | - | - | - |
M2BA | S. warneri | Mascara | 0 | - | - | - | |
M2BB | S. hominis ssp. hominis | Mascara | 0 | - | - | - | |
EY5 | S. xylosus | Eyeliner | 0 | - | - | - | |
EY6 | S. warneri | Eyeliner | 0 | - | - | - | |
C7 | S. aureus | Concealer | BEN/ OX/ TET | 2 | - | + | - |
SgA2 | S. epidermidis | Shower gel | BEN/ TET | 2 | - | - | - |
Isolate Origin | Species | Biofilm Formation-encoding Genes | ||||
---|---|---|---|---|---|---|
icaA | icaB | icaC | icaD | icaR | ||
PW10 /face powder | S. haemolyticus | + | + | + | + | + |
SgA2/shower gel | S. epidermidis | - | - | - | - | - |
LP27/lipstick | S. epidermidis | + | + | + | + | + |
BLA/blusher | S. epidermidis | + | + | + | - | - |
BLB/blusher | S. epidermidis | - | - | - | - | - |
C7/concealer | S. aureus | - | - | - | - | - |
BLBc/blusher | S. epidermidis | - | - | - | - | - |
LP24/lipstick | S. aureus | + | + | - | + | + |
Total | 8 | 4 (50) |
4 (50) |
3 (37.5) |
3 (37.5) |
3 (37.5) |
Isolate Origin | Species | Methicillin–resistance Gene |
---|---|---|
mecA | ||
PW10/face powder | S. haemolyticus | + |
SgA2/shower gel | S. epidermidis | - |
LP27/lipstick | S. epidermidis | - |
BLA/blusher | S. epidermidis | - |
BLB/blusher | S. epidermidis | - |
C7/concealer | S. aureus | + |
BLBc/blusher | S. epidermidis | - |
LP24/lipstick | S. aureus | + |
Total | 8 | 3 (37.5) |
3.2. Molecular Detection of Biofilm Formation and Methicillin Resistance-encoding Genes
PCR analysis detected the biofilm formation-linked genes in eight selected Staphylococcus spp. isolates in cosmetic samples. All the tested Staphylococcus spp. isolates possessed icaR, icaD, icaC, icaB, and icaA encoding genes (Table 7 and Fig. (1A-E). The icaA and icaB genes were detected in 50% (four isolates), whereas the prevalence of the icaC, icaD, and icaR genes was 37.5% (three out of eight isolates) (Table 7). Three isolates of S. haemolyticus, S. aureus, and S. epidermidis tested positive for all the ica genes (Table 7). The methicillin resistance-encoding gene (mecA) was detected in only three of the eight tested isolates (Table 8 , Fig. 2).
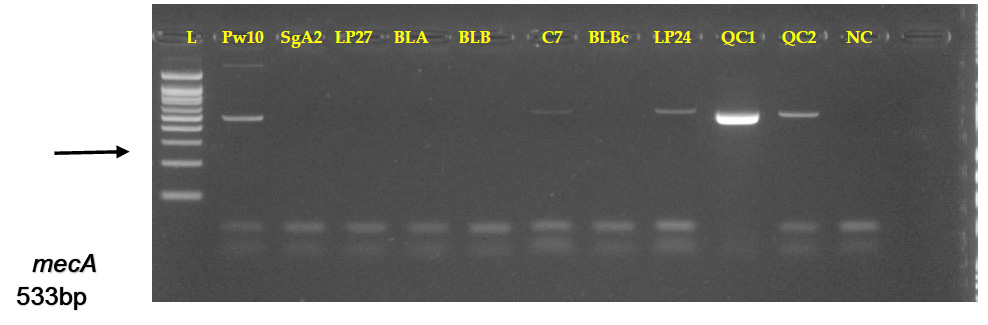
4. DISCUSSION
The usage of cosmetic products has become a necessary daily routine in recent times [2]. Consumers are turning towards biologically derived beauty products, which has facilitated significant growth of the global cosmetics market [21]. Cosmetic production often utilizes non-sterile raw materials, however, they are considered safe if bacterial counts remain within the permissible limit. For example, the bacterial count in eye-related cosmetics should remain below 500 CFU/g, whereas 1000 CFU/g is the maximum limit for other cosmetics of 1 g or 1 mL size [22]. The sterilization of cosmetic products is not carried out even though they might contain infectious bacteria. However, cosmetic products should be free from pathogens such as Pseudomonas aeruginosa, Staphylococcus aureus, and Escherichia coli. Moreover, maintaining a lower microbial count is mandatory [4].
Staphylococcus spp. are a common part of skin microbiota that justifies their frequent detection in cosmetics. However, certain Staphylococcus spp. isolates can be pathogenic and cause skin infections [23]. This study thoroughly examined the diversity, antimicrobial susceptibility patterns, and biofilm formation of Staphylococcus spp. in cosmetic products of various qualities in Western Saudi Arabia. The results revealed Staphylococcus spp. contaminations in cosmetic products. A total of 250 samples were analyzed, and 26 samples emerged positive for Staphylococcus spp. Most Staphylococcus spp. were detected in the eyes and lips-related cosmetic products. These results are in line with the findings of Nasir and Qasim [24], who also observed the highest Staphylococcus epidermidis concentrations in eyeliners and lipsticks in Iraq. Alshehrei [25] also reported that S. epidermidis and S. aureus were prominent Gram-positive species in eyeliner, lipstick, and lip-gloss products of Makkah, Saudi Arabia.
This study further confirms previous findings by detecting significant bacterial levels of different Staphylococcus species in lipstick and eyeliner, including the pathogenic S. epidermidis and S. aureus . The favorable conditions in moist cosmetic products may contribute to the higher levels of Staphylococcus spp.The prevalence of pathogenic Staphylococcus spp. in eyeliners could result in eyelid inflammation and blepharitis [26].
A higher prevalence of Staphylococcus spp. was noted in non-branded cosmetics (low-quality/counterfeit products). In addition to lipstick and eyeliners, other low-quality products (mascara, blushers, face powders, shower gels, and concealers) from traditional local markets have also tested positive for different pathogenic Staphylococcus spp. (S. haemolyticus, S. aureus, and S. epidermidis) [27]. Less pathogenic species (S. homins and S. warneri) were also detected in low-quality cosmetics. However, recent studies have reported opportunistic infections of these less pathogenic species in immunocompromised individuals [28]. Fatima [29] also reported the presence of S. haemolyticus and S. hominis in cosmetic products. The prevalence of potentially pathogenic Staphylococcus spp. in low-quality or counterfeit cosmetics indicates poor manufacturing, handling, storage, and transportation conditions. Nusrat et al. [23] investigated microbial contamination in local cosmetics of Bangladesh and detected pathogenic bacteria (Klebsiella pneumoniae, Staphylococcus spp., Pseudomonas aeruginosa, Streptococcus spp., and Salmonella spp.) in more than 80% of tested products. They attributed these contaminations to poor manufacturing practices, storage and handling, and low-quality ingredients. Low-quality cosmetics might also contain hazardous ingredients, which could damage the skin and disrupt the normal skin microbial flora, leading to bacterial infections [30]. Thus, low-quality cosmetic products from unknown manufacturers should not be utilized to avoid potential pathogenic bacterial contaminations.
Antimicrobial susceptibility profiles of detected Staphylococcus spp. revealed a low prevalence of MDR strains in cosmetic products. S. xylosus was the only MDR species, which is not associated with human infections. Nonetheless, potentially pathogenic S. haemolyticus, S. aureus, and S. epidermidis isolates were resistant to tetracycline, benzylpenicillin, erythromycin, and oxacillin, which is in line with previous studies [31, 32]. Tetracycline and erythromycin are often administered against mild skin infections of S. aureus. However, the emergence of MRSA strains and tetracycline and erythromycin-resistant S. aureus strains have complicated their treatment [17]. Tetracycline and erythromycin resistance have also been reported in S. haemolyticus and S. epidermidis in nosocomial infections [16, 33]. The results revealed antibiotic resistance among less pathogenic Staphylococcus spp. including S. xylosus, S. hominis, S. lentus, S. warneri, and S. sciuri. These species demonstrated resistance against rifampicin, oxacillin, tetracycline, and clindamycin. Thus, these species might serve as reservoirs of resistance genes, which could spread to other clinical biofilm-forming staphylococci communities via horizontal gene transfer [34].
Biofilm-forming Staphylococci spp. are associated with chronic infections, particularly related to implanted medical devices [35]. S. epidermidis was reported as the first biofilm-producing species. Then, later studies reported biofilm formation in S. aureus and other CoNS (coagulase-negative staphylococci) as well [36]. Biofilms are exopolysaccharide matrix-surrounded bacterial aggregates on a surface [37]. The biofilm-forming capability enables inherent bacterial resistance to different antimicrobial drugs and the host’s immune responses [38]. The results of this study depicted the presence of biofilm-encoding genes (icaA and icaB) in 50% of the examined (n =8) Staphylococcus spp. (S. haemolyticus, S. aureus, and S. epidermidis). The same isolates also contained the icaC gene (S. epidermidis), icaR and icaD genes (S. aureus), and icaR, icaD, and icaC genes (S. haemolyticus and S. epidermidis). These isolates exhibited resistance to at least one antibiotic. It establishes a potential link between multidrug resistance and the biofilm formation capability of bacteria [21, 39-43].
This study highlights the public health concerns of Staphylococcus spp. contamination in cosmetic products in Western Saudi Arabia. The presence of MRSA and antibiotic-resistant biofilm-forming S. haemolyticus and S. epidermidis in cosmetic products suggest potential pathogenic transmission to consumers. Particularly, antibiotic-resistant bacteria (S. haemolyticus, S. aureus, and S. epidermidis) constitute serious health concerns as their treatments are complicated and thus might yield serious outcomes. The carriage of biofilm formation-encoding genes by S. haemolyticus, S. aureus, and S. epidermidis further aggravates the situation. The biofilm-forming bacterial communities exert more resistance to antimicrobial agents, and these bacteria could acquire resistance-encoding genes via horizontal gene transfer. Therefore, the current study emphasizes strict regulation measures regarding the manufacturing, storage, and handling of cosmetics. The remedial measures could alleviate consumers’ infection risks by preventing contaminated cosmetics-associated outbreaks.
5. STUDY LIMITATIONS
The current study comprehensively elaborates on various aspects of Staphylococcus spp. (prevalence, diversity, antimicrobial resistance, and biofilm formation) in cosmetic products in Western Saudi Arabia. However, the following limitations should be considered as well.
- ● Sample size: A total of 250 cosmetic samples were analyzed in this study, revealing Staphylococcus spp. contamination in 26 samples (10.4%) of the selected cosmetic products. However, analyzing a larger sample size of various cosmetic products across different geographic regions could provide a broader perspective and further validate the findings of this study.
- ● Geographic limitation: The study primarily focused on Western Saudi Arabia, limiting the applicability of its findings to regions with different regulatory, manufacturing, and environmental conditions. Future studies should conduct national or multinational assessments to compare regional contamination rates in cosmetic products.
- ● Product classification: The study compared branded (well-known brands) and non-branded (counterfeit and unknown) cosmetics, observing higher contamination rates in low-quality or counterfeit products. However, it lacks a systematic analysis of ingredient sources, as well as manufacturing and storage conditions. Future studies should further explore the role of these factors in microbial contamination.
- ● Longitudinal analysis: This study only demonstrates Staphylococcus spp. contaminations in cosmetic products at a specific time. A future longitudinal study could reveal contamination variations over time in correlation with cosmetic products’ shelf life, user habits, and storage conditions.
- ● Molecular and genomic characterization: This study identified the mecA and biofilm formation-associated genes. However, the application of the whole-genome sequencing (WGS) technique in the future could facilitate detailed characterization of virulence factors, resistance genes, and potential horizontal gene transfer (HGT). Future investigations should also consider incorporating next-generation sequencing (NGS) for a detailed understanding of staphylococci resistance-related genetic mechanisms in cosmetic products.
6. RECOMMENDATIONS
The study highlights the significant public health risks associated with cosmetic contamination. The presence of Staphylococcus spp., along with their antimicrobial susceptibility profiles and biofilm-forming capacity, further exacerbates the concern. The following recommendations may help mitigate these risks. Industrial scale regulatory measures:
Implementation of strict microbiological safety regulations during the manufacturing, storage, and distribution of cosmetic products is necessary to mitigate microbial contaminations. Regular microbial testing of cosmetic formulations should be mandatory to restrict bacterial count within judicial limits, particularly in lip and eye products. Potential contamination risks and recommended duration should be clearly labeled on the cosmetic products.
6.1. Surveillance and Antimicrobial Stewardship Program
Antibiotic resistance patterns of cosmetic-contaminating bacteria should be regularly monitored via an integrated surveillance system. It should particularly include the surveillance of methicillin-resistant Staphylococcus aureus (MRSA). The collaboration of manufacturers, regulatory agencies, and healthcare authorities could help in the rapid evaluation of emerging antimicrobial resistance patterns in cosmetic products. Moreover, antimicrobial alternatives should be screened to restrict microbial contaminations in cosmetic products without exerting resistance selection pressure.
6.2. Consumer Awareness and Hygiene Practices
Consumer awareness is important regarding proper cosmetic handling and storage. Precautionary measures should include the usage of clean application tools, avoiding product sharing, and discarding expired products. Low-quality/counterfeit cosmetic products-related potential health risk awareness should be enhanced. The products manufactured without appropriate regulatory approval should be particularly focused. Additionally, innovative hygienic packaging such as antimicrobial applicators, airless pumps, and single-use cosmetic samples should be promoted to alleviate contamination risks.
7. FUTURE DIRECTIONS
The study emphasizes further investigations regarding bacterial prevalence, emergence of resistance, and contamination reduction strategies in cosmetic products.
7.1. Antimicrobial resistance mechanisms
Whole-genome sequencing and transcriptomic investigations could elucidate the mechanisms of antimicrobial resistance gene acquisition and transmission in cosmetics-associated Staphylococcus spp. Resistant bacterial isolates should be examined for biofilm formation-linked genes and efflux pump activity to enhance the understanding of bacterial resistance mechanisms in cosmetic formulations against antimicrobial agents. Cosmetic preservatives’ efficiency
Current cosmetic preservatives should be evaluated against multidrug-resistant (MDR) Staphylococcus spp. Novel antimicrobial agents (synthetic and natural), such as bio-based preservatives, essential oils, and nanoparticles, should be assessed as safer alternatives to traditional preservatives.
7.2. RISK FACTORS
Raw material sources, production conditions, and supply chain logistics should be assessed and monitored to avoid microbial contaminations. Moreover, metagenomics-based investigations of the entire microbial community in cosmetic products could provide insights into detailed microbial ecology.
7.3. Human health implications of cosmetic-associated Staphylococcus spp.
Clinical studies are necessary to estimate infection risk from contaminated cosmetic products, particularly in immunocompromised individuals. The cosmetics should also be investigated in correlation with Staphylococcus spp. related skin conditions such as periocular infections, acne, conjunctivitis, and folliculitis
CONCLUSION
This study establishes cosmetic products as a significant medium for pathogenic microorganisms’ transmission to humans. Particularly, the transmission of antibiotic-resistant S. aureus is seriously detrimental to human well-being. Moreover, the study correlates antibiotic resistance in staphylococci with their biofilm-forming capability. Collectively, the investigation emphasizes the implementation of health regulations at all stages of cosmetics production. Furthermore, it suggests the inclusion of preservatives without compromising human health while simultaneously hindering microbial growth during the manufacturing, preservation, transportation, and marketing of cosmetics. The insightful findings emphasize further large-scale investigations, genomic analyses, and clinical investigations for efficient microbial alleviation strategies. The collaboration of regulatory agencies, cosmetic producers, and healthcare professionals could ensure consumer safety by reducing antibiotic-resistant bacterial transmissions from everyday cosmetic products.
AUTHORS’ CONTRIBUTION
Conceptualization: HHA; Methodology: HHA, FMAb; Investigation: MSAh, HHA, SAg; Data curation: MSAh, HHA, LAN, FMAb; Formal analysis: FMAb, RAAg, LAN; Resources: HHA; Writing – original draft preparation: FMAb; Writing – review and editing: HHA, LAN; Supervision: FMAb.
All authors have read and agreed to the published version of the manuscript.
AVAILABILITY OF DATA AND MATERIAL
All the data and supporting information are provided within the article.
ACKNOWLEDGEMENTS
Declared none.