All published articles of this journal are available on ScienceDirect.
An Evaluation of Bacterial Inhibition on E-max and Zirconia Disks after Different Surface Treatments: A Comparative In vitro Study
Abstract
Background
Comprehending how bacteria adhere to different materials is essential for ensuring the long-term success of dental restorations. Various types of ceramic dental materials with distinct chemical and mechanical properties have been developed for this purpose. Therefore, this in vitro study aimed to investigate bacterial inhibition on E-max and zirconia disks after different surface treatments.
Materials and Methods
Forty discs of zirconia and 40 discs of lithium disilicate-pressed E-max were prepared with standardized dimensions. Each main group was divided into two subgroups (20 for each). One subgroup was subjected to polishing and glazing, while the second one was not. After thermocycling, discs in each subgroup were placed on the bacteria culture plates containing either Streptococcus mutans (S. mutans) or Escherichia coli (E. coli).
Results
The zirconia polishing group achieved the greatest bacterial inhibition, followed by the polished E-max group. The glazed zirconia and glazed E-max groups had lower values and highly significant differences were found.
Conclusion
Bacteria were more inhibited in the polished groups than in the glazed groups of both zirconia and E-max.
1. INTRODUCTION
The oral cavity consists of a unique environment for the formation of complex biofilms. To survive within the oral cavity, bacteria must adhere to either soft or hard tissues to withstand shear forces. In addition to the hard tissues of teeth, the oral cavity also contains commonly used dental restorative materials with hard surfaces to which bacteria can adhere, such as ceramics [1]. The restorative materials used in the oral cavity should possess surface properties that prevent bacterial adhesion and biofilm formation.
Quantitatively understanding bacterial adherence to various materials is crucial for ensuring the long-term success of dental restorations [2, 3]. A range of ceramic dental materials with diverse chemical and mechanical properties have been developed. Among these, lithium disilicate and zirconia ceramics are noteworthy. These materials offer outstanding mechanical and chemical properties, such as biocompatibility, a propensity to minimize plaque buildup, and favorable interactions with soft tissues, making them ideal for the gingival prostheses interface [4]. Oral biofilms are well-characterized microbial systems that form in two stages. Initially, specific bacteria adhere to the pellicle, which is followed by the accumulation of additional bacteria. The bacteria involved in the early stages of biofilm attachment are known as “early colonizers” and are typically facultative anaerobes. For example, Streptococcus mutans is one of the first bacteria to colonize initial supra-gingival biofilms within the first 8 hours and is found in higher quantities in oral biofilms, while secondary colonization occurs 3 to 5 days after the acquired pellicle begins to form, and the biofilm reaches maturity in 2 to 3 weeks [1, 5]. The adhesion of bacteria to a surface and the initial composition of the biofilm are influenced by various factors, including mass transport, interactions between existing microorganisms, surface conditioning, surface charge, hydrophobicity, surface roughness, and microtopography. These factors can all contribute to the formation of biofilms. On hydrophilic surfaces, a water layer can form, which makes it challenging for hydrophobic microorganisms to make direct contact with the substrate [6, 7]. Among these factors, the impact of surface roughness on bacterial attachment has been widely studied, but the findings are inconsistent. Techniques for finishing and polishing can affect both the roughness and mechanical properties of zirconia. Furthermore, the smoothness of the restoration surface directly influences bacterial adhesion [8]. Over the last ten years, scientific research on dental ceramics has focused on zirconia and lithium disilicate. Ceramic systems are, therefore, subject to constant revision and updating to incorporate new ceramic materials [1].
Zirconia surfaces are less homogeneous compared to other materials due to acquiring pores from the sintering process and defects from polishing. These imperfections arise because of the larger grains in zirconia, which are prone to exposure during the polishing process [6, 9, 10].
The all-ceramic IPS e.max® system (introduced in 2005), composed of lithium disilicate, has established new benchmarks for optical and mechanical performance. Lithium disilicate is a glassy ceramic known for its average flexural strength of 400 MPa and favorable translucency, making it appropriate for both anterior and posterior applications. It offers several advantages over conventional metal materials, macromolecular materials, and older ceramics, including high mechanical and flexural strength, excellent wear resistance, and superior aesthetics [11]. Glazed ceramic restorations not only enhance the natural appearance of dental prosthetics, but also improve their inert properties. The glaze provides a vitrified layer that seals the pores of the ceramic’s surface, which helps to prevent the penetration of bacteria and oral fluids. It is composed of a colorless glass powder that is melted over the last superficial layer, producing a light reflection similar to that of natural teeth. However, the glaze material gets worn off in a short period of time [12, 13]. To restore the polish on ceramic restorations, dentists can choose between reglazing or using polishing burs. Reglazing must be done in a laboratory, requiring an additional appointment to complete the restoration. Additionally, a second glaze firing may lead to negative effects on the ceramic, such as devitrification. In contrast, polishing systems can be performed chairside in a single clinical session [13]. Ceramic polishing kits ensure a smooth surface, provide durable results, and are cost-effective. Additionally, the polishing process is straight forward [14].
Biofilms are complex microbial communities typically connected to a surface and embedded in a 3D extracellular matrix consisting of proteins, exopolysaccharides, and extracellular DNA; during the early stages of biofilm development, bacterial attachment to solid surfaces may be influenced by the electrical charges of both the bacteria and the substrate. Since bacterial surfaces typically carry a negative charge, contact with a positively charged zirconia surface can create an electrostatic imbalance, potentially disrupting the cell membrane potential and leading to physiological damage and preventing bacterial adhesion [15, 16]. To avoid biological issues, like secondary caries or periodontal disease, following prosthetic restorative treatment, it is crucial to examine and understand the attachment of early-colonizing bacteria to restorative materials. Bacterial adherence is the key step in the formation and development of oral biofilms. The objective of this in vitro study was to evaluate and compare the antibacterial effect of zirconia versus lithium disilicate after using two finishing systems (polished and glazed). In relation, the null hypothesis was that there exists no significant difference between zirconia and lithium disilicate as well as between the polished and glazed specimens of each type.
2. MATERIALS AND METHODS
2.1. Sample Preparation of the Zirconia Discs
A rectangular cubic shape was generated from the pre-sintered Y-TZP (ELEMENT Z-zircon blanks) zirconium oxide blocks, and then the rectangular cubic shape was longitudinally divided into three rectangular cubic bars with dimensions of 6.5-7.0 mm in diameter and 3.0 mm in thickness.
The central point at one end of each zirconia rectangular cubic bar was identified as the reference for fitting the bar with its long axis aligned for milling. This process involved modifying the rectangular cubic bar into a cylindrical shape with a uniform diameter of 8.0 mm. The fitting bar was adapted to a low-speed rotating machine, and milling was performed longitudinally using a laboratory micromotor with a straight headpiece. This setup allowed for free movement along the zirconia blank's side, transforming the rectangular bar into a cylindrical one with a consistent diameter. Once the milling was complete, sandpaper was used to smooth the cylindrical bar shape through longitudinal movements, ensuring it met the required specifications.
The zirconia cylindrical bars were sliced into discs by cutting perpendicular to the bar using a carbide disc, thereby achieving a consistent thickness of 3 mm. These discs were then sintered in a tube furnace (HT-S MV MIHMVOGT-Germany 2014) at 1500 °C for 8 hours, followed by cooling according to the manufacturer's instructions. During this process, the discs underwent a 25% volumetric shrinkage, resulting in the milled cylinders with 25% more volume initially. After sintering, the discs were measured to have dimensions of 7 mm in diameter and 3 mm in height.
Subsequent to sintering, 20 discs were finished, polished, and glazed according to the manufacturer's recommendations, forming the “zirconia glazed” (ZG) group. Another set of 20 discs, referred to as “zirconia polished” (ZP), were polished with 120-grit emery paper and a felt wheel with diamond paste (Dia-Finish, Renfert GmbH, Hilzingen, Germany), but were not glazed.
2.2. Sample Preparation of the Lithium Disilicate Discs
The ingots of the IPS e.max press were cut by straight hand through diamond-cutting disc sides with copious water cooling in a direction perpendicular to the ingot, and then the discs were finished, polished, sintered, and ground according to the manufacturer’s recommendations for 20 discs. This group was called E-max glazed (EG), and the other 20 discs were not glazed. The specimens were polished with 120-grit emery paper (15 disks and a felt wheel with diamond paste (Dia-Finish, Renfert GmbH, Hilzingen, Germany), and were called E-max polished (EP).
Two of the authors evaluated the samples for visible cracks or scratches using 2.5× optical magnification (SurgiTel, USA). The discs were then subjected to 1000 thermal cycles between 5 and 55°C, after which the sample was incubated in a thermal bath for 20 seconds at each temperature according to the International Organization for Standardization, after which the bacterial suspension was added.
2.3. Ethical Approval
Ethical approval was obtained from the Kufa University/Najaf, Iraq (reference number: DEC-36), and consent was secured from all patients and their guardians. Additionally, all participants were verbally informed about the study, and their consent for both the experiments and publication of the results was obtained before sample collection.
2.4. Rationale and Study Design
Bacterial adhesion to dental restorative materials significantly impacts restoration longevity. This in vitro study directly compared bacterial inhibition on zirconia and E-max disks, evaluating the effects of polishing and glazing, two common surface finishing techniques. The goal was to provide clinicians with evidence-based guidance for material selection and surface treatments to minimize biofilm formation.
Forty disks each of zirconia (ELEMENT Z-zircon blanks, yttrium-stabilized) and lithium disilicate (IPS e.max press LT B1) were prepared (n = 40 per material, total n=80). This sample size was determined based on a priori power analysis using G*Power software (version 3.1.9.7). We aimed to detect a medium effect size (f = 0.25) with a power (1 - β) of 0.80 and a significance level (α) of 0.05 for one-way ANOVA analysis. The power analysis indicated that a total sample size of 76 (19 per group) would be sufficient [17, 18]. We chose n=20 per group (and thus 40 per material) to provide a slightly larger sample size, further enhancing statistical power. Each material group was subdivided into polished and glazed groups (n=20 per subgroup). Supra-gingival plaque samples, used for Streptococcus mutans and Escherichia coli culture, were collected from 40 patients attending the clinic. Patient selection excluded those with chronic systemic diseases, pregnancy, smoking, antibiotic/anti-inflammatory use within the past 3 months, or recent professional cleaning/periodontal treatment (within the last 6 months). Patients were aged 30-55 years. Polishing and glazing were selected as clinically relevant surface treatments. S. mutans was chosen as a primary cariogenic oral bacterium, and E. coli as a model gram-negative bacterium.
2.5. Bacterial Sample Preparation
The supra-gingival plaque samples were collected from 80 patients (aged 30-55 years) attending the clinic. Inclusion criteria were participants aged between 30 and 55 years and attending the University of Kufa dental clinic. This ensured that the participants were available for sample collection at the clinic. Exclusion criteria included those with any chronic systemic disease, being pregnant, smoking, or using antibiotics and/or anti-inflammatory drugs in the past 3 months, and undergoing professional cleaning or periodontal treatment within the last 6 months. The plaque was placed on a swab that was inserted immediately into transfer media to preserve the sample, which was then spread on selective agar media for both S. mutans and E. coli. The procedure was performed within a period of less than 30 minutes after sample collection.
Each bacterial strain was cultured under its specific conditions; S. mutans and E. coli were grown in brain-heart infusion (Bacto Brain Heart Infusion, Becton, Dickson and Company, MD, USA) and Luria–Bertani (LB) broth (LB-Medium, MP Biomedicals, CA, USA), respectively, at 37 °C for 24 hours in ambient air. The optical densities of the bacterial suspensions were measured at 600 nm using a UV-visible spectrometer (V-550, JASCO, Tokyo, Japan) to achieve concentrations ranging from 0.4 to 3.0 × 10^8 colony-forming units (CFU)/mL. S. mutans was identified using the GP-ID VITEK-2 compact, while E. coli was identified using the GO-ID VITEK-2 compact.
Following the manufacturer's instructions, 3 ml of normal saline was added to a test tube and inoculated with a loopful of isolated colonies. The test tube was then placed in a densitometer to standardize the colony to a McFarland standard solution (1.5 x 10^8 cells/mL). The standardized inoculum was loaded into a cassette, and a sample identification number was entered into the computer software via barcode. The VITEK-2 card type was read from the barcode on the card, linking it to the sample ID number. The cassette was then placed in the filling module, and once the cards were filled, the cassette was moved to the reader/incubator module and the test data were transferred to the computer for analysis.
2.6. Antibacterial Test
The antibacterial test was performed using the disk diffusion method (Fig. 1) to evaluate the antibacterial activity of zirconium and E-max. This was assessed by measuring the zone of inhibition (in mm) against S. mutans and E. coli.
Before performing the antibacterial test, all samples were disinfected with 70% ethanol, rinsed with distilled water, and then dried [19]. Bacterial suspensions were applied to the samples, which were then covered with sterilized plastic film and incubated at 37 °C for 24 hours. Fig. (1) represents a summary of the grouping performed in this study. Samples were placed on bacterial culture plates and incubated at 37 °C for 24 hours. The antibacterial activity was determined by calculating the average size of the inhibition zone around the disks on the agar plates. Each test was conducted in triplicate, and the effectiveness was measured by assessing the clear zone of inhibition around the disks using a Vernier caliper [20].
2.7. Statistical Analysis
The results were subjected to statistical analysis and analyzed using Microsoft Excel and SPSS-28 (a statistical package for social sciences, version 24). The results have been expressed as mean ± SD (standard deviations). One-way analysis of variance (ANOVA) and Fisher’s least significant difference (LSD) tests were employed to compare the differences among the studied materials, with p≤0.05 indicating the lowest limit of significance.
3. RESULTS
The polished zirconia (ZP) surface exhibited the highest inhibition effect against Streptococcus mutans (2.00±0.76), followed by polished E-max (0.60±0.29), with a significant difference (P <0.01) (Table 1; Figs. 2 and 3). On the other hand, the same result was observed against E. coli bacteria, with only the polished zirconia (ZP) and polished E-max (EP) surfaces exhibiting significant inhibition effects (Table 2; Figs. 4 and 5).
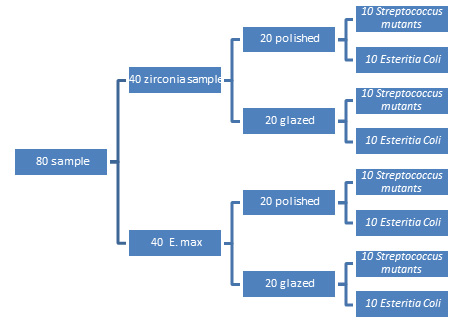
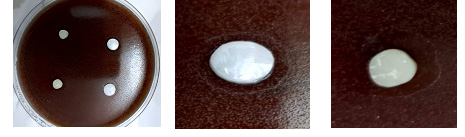
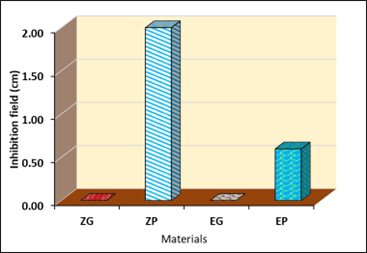
Material | Mean±SD | P-value | Multiple Pairwise Comparison Mean Difference (P-value) |
|||||
---|---|---|---|---|---|---|---|---|
ZG viz. ZP | ZG viz. EG | ZG viz. EP | ZP viz. EG | ZP viz. EP | EG viz. EP | |||
ZG | 0.00±0.00a | <0.01* LSD=1.12 |
||||||
ZP | 2.00±0.76b | -2.0 (<0.01) |
||||||
EG | 0.00±0.00a | 0.000 (1.000) |
2.0 (<0.01) |
|||||
EP | 0.60±0.29a | -0.60 (0.232) |
1.40 (<0.01) |
0.60 (0.232) |
Material | Mean±SD | P-value | Multiple Pairwise Comparison Mean Difference (P-value) |
|||||
---|---|---|---|---|---|---|---|---|
ZG viz. ZP | ZG viz. EG | ZG viz. EP | ZP viz. EG | ZP viz. EP | EG viz. EP | |||
ZG | 0.00±0.00a | <0.01* LSD=1.25 |
||||||
ZP | 1.80±0.62b | -1.8 (<0.01) |
||||||
EG | 0.00±0.00a | 0.000 (1.000) |
1.8 (<0.01) |
|||||
EP | 0.36±0.30a | -0.36 (0.119) |
1.44 (<0.01) |
0.36 (0.119) |
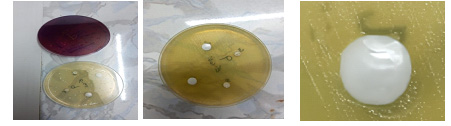
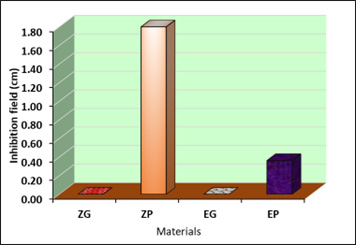
4. DISCUSSION
This in vitro study investigated the effects of glazing and polishing on the antibacterial effects of CAD/CAM-milled zirconia and lithium disilicate-pressed E-max. The study utilized ceramic materials that were ELEMENT Z-zircon blanks, including new yttrium-stabilized zirconia (ZrO2) and aluminum oxide (Al2O3) with fine grain sizes for smooth surfaces and high biocompatibility, and IPS e.max press LT B1, which is a low-translucent ingot ideally processed using the cut-back technique as well as the staining technique. Both of these materials have been recently introduced to the dental market, and these products have not yet been examined for their surface roughness and bacterial adhesion. The VITA AKZENT® Plus glaze is suitable for producing glass-like surfaces. Various polishing kits were utilized for each ceramic type, following the manufacturers' recommendations. In our in vitro study, we employed Streptococcus mutans because it is a key cariogenic bacterium, significantly increasing the pathogenicity of biofilms [21, 22].
The bacterium E. coli is not very often found in the oral environment, but it was collected from our swab samples. In this study, it was primarily utilized for comparison as it is a widely recognized model for Gram-negative bacteria [23]. According to Thomas Thurnheer and Georgios N, the growth of E. coli can be intensified and observed as a pervasive pattern throughout the biofilm. The disks were 6.5-7 mm in diameter and 3 mm thick. The size selection was based on the requirements of the antibiotic office, which specified that the pieces should be circular with a diameter of 6.5–7 mm, as the smaller the pieces are, the stronger the result. A larger area indicates the elimination of bacteria, and it is more difficult to measure the inhibition zone. Based on the statistical analysis of the results, the zirconia polished group showed greater inhibition field values for E. coli and Streptococcus mutans than did the glazed group. Oral bacterial adhesion can be categorized into four stages, i.e., bacterial movement toward the surface, early adhesion that can be reversible or irreversible, adhesion through specific interactions, and the formation of a biofilm [17, 24].
In a healthy condition, bacterial adhesion and removal from the oral surface maintain a dynamic balance. However, when bacterial accumulation increases for various reasons, it can lead to disease. Factors influencing the extent of bacterial adhesion include surface roughness and surface free energy (SFE). An increase in surface roughness on an irregular surface boosts bacterial adhesion by creating sheltered areas within the surface irregularities. Additionally, surfaces with high energy attract more bacteria and facilitate stronger bonding [16]. The impact is selectively enhanced in the case of specific bacteria, and these two factors interact in a way that often counterbalances each other's effects. According to Al-Radha et al., the low surface energy of zirconia diminishes bacterial adhesion and directly influences the dental pellicle formed by saliva [25]. In this study, increased colony formation was noted on glazed surfaces compared to polished surfaces, supporting earlier research findings. The application of glaze led to rougher surfaces across all ceramic materials. This roughness in glazed types could be due to the chemical bond between the glaze and materials, like zirconia and E-max, where the glaze tends to accumulate in patches. This aligns with other studies that have found polished surfaces to be smoother and have lower surface free energy. Zirconia’s reduced bacterial adhesion may be likely due to its lower surface free energy, as when surface energy rises, the bacterial adhesion also increases [6, 17, 26, 27].
However, the current study was not in accordance with the findings of Shirin Lavaf et al., who proved bacterial adhesion to be highest on non-glazed surfaces, while over-glazed surfaces showed the least adhesion. The glazed surfaces exhibited more inhibition than the polished surfaces [28]. The polished E-max group had higher bacterial inhibition than the glazed group, possibly because the differences in adherence were due to the variations in roughness values as well as the fabrication and surface modification factors rather than differences in crystal size [29]. In general, the reason for bacterial inhibition may be attributed to the absence of necessary receptors on the sample surface for initiating early attachment for biofilm formation or changes in pH and releasing some elements toxic to bacteria. This indicates that zirconium and E-max not only inhibited bacterial adhesion, but also exhibited antibacterial effects, being in line with a previous study [17]; however, further studies are needed to study the components of media after sample insertion.
5. LIMITATIONS
This study involved several limitations. Firstly, the in vitro design may have been a constraint because it could not accurately replicate the oral environment, differing from actual intraoral conditions. Additionally, finishing and polishing of the specimen were limited to a single protocol, and the effects of tooth brushing and saliva on bacterial cleaning were not considered. Secondly, the actual intraoral biofilms are heterogeneous, consisting of various microorganisms; however, this study included only two types of microorganisms, S. mutans and E. coli. Lastly, the study's statistical analysis was limited to a small number of samples, and a larger sample could provide more reliable results and greater statistical power.
CONCLUSION
There were significant differences in bacterial inhibition, which may be influenced by the material composition. Relatively greater bacterial inhibition was observed on zirconia surfaces compared to lithium disilicate.
The finishing process affects bacterial inhibition by altering surface free energy and roughness. Glazed surfaces (zirconia and lithium disilicate), in contrast to mechanical polishing, resulted in lower surface roughness and minimal morphological alterations in the polished groups, and thus greater bacterial inhibition. This study included only two types of microorganisms, S. mutans and E. coli; therefore, further research involving a broader range of bacterial strains found in the oral cavity is necessary to investigate the synergistic effects on bacterial adhesion and inhibition. Drawing definitive conclusions from the reported results can be challenging. The composition of materials can impact the initial adhesion of bacteria; thus, further research is necessary to determine whether there is a correlation between bacterial inhibition and the glass content in ceramics. Moreover, gaining a deeper understanding of the mechanisms behind bacterial inhibition and adhesion to different materials used in oral rehabilitation is essential.
REGULATORY STATEMENT
This article did not use hazardous materials, living organisms, or procedures that could potentially harm the environment. No specific regulatory laws or regulations related to occupational health and safety or environmental protection were required. All appropriate measures were taken to ensure adherence to ethical research practices and laboratory safety.
AUTHORS’ CONTRIBUTIONS
It is hereby acknowledged that all authors have accepted responsibility for the manuscript's content and consented to its submission. They have meticulously reviewed all results and unanimously approved the final version of the manuscript.
LIST OF ABBREVIATIONS
LSD | = Least significant difference |
ZP | = Polished zirconia |
CFU | = Colony-forming units |
ZG | = Zirconia glazed |
ETHICS APPROVAL AND CONSENT TO PARTICIPATE
Ethical approval was obtained from the Kufa University, Najaf, Iraq (reference number: DEC-36).
HUMAN AND ANIMAL RIGHTS
All procedures performed in studies involving human participants were in accordance with the ethical standards of institutional and/or research committee, and with the 1975 Declaration of Helsinki, as revised in 2013.
CONSENT FOR PUBLICATION
Consent was secured from all patients and their guardians. Additionally, all participants were verbally informed about the study, and their consent for both the experiments and publication of the results was obtained before sample collection.
AVAILABILITY OF DATA AND MATERIALS
The data and supportive information are available within the article.
ACKNOWLEDGEMENTS
The authors thank Dr. Julfikar Haider, the Department of Engineering, Manchester Metropolitan University, Manchester M15 GD, UK, for his valuable contributions and advice.