All published articles of this journal are available on ScienceDirect.
Synergistic Effects of Antibiotic Combinations against Staphylococcus aureus in Clinical Samples from Inpatients at a Tertiary Care Facility in Hyderabad, India
Abstract
Background
Staphylococcus aureus infections are commonly caused by bacteria in community and hospital settings. The basis of modern medicine is at risk due to the worldwide problem of antibiotic resistance and the need to discover viable antimicrobials. One way to tackle antibiotic resistance (AMR) is by combination therapy. Due to their promising efficacy against bacteria, the current research focuses on combining antibiotics.
Objective
This study aimed to study the synergistic effects of antibiotic combinations against Staphylococcus aureus from clinical samples of inpatients at a tertiary care hospital in Hyderabad, India
Materials and Methods
Staphylococcus aureus (S. aureus) was isolated from the in-house clinical samples and was further subjected to antimicrobial susceptibility testing. Minimum Inhibitory Concentration (MIC) by broth microdilution method was determined against four antimicrobials. Biofilm formation and time-kill analysis were performed for combination antibiotics. The checkerboard test for fractional inhibition (FIC) was used to evaluate the synergistic effect of both agent combinations.
Results
A total of 3663 clinical samples, with 185 identified as S. aureus. Methicillin-resistant S. aureus (MRSA) were confirmed by phenotypic and genotypic methods showing positive for 84 isolates (45%). The prevalence was high in male patients at 51% and in the critical care wards at 30%, and blood samples scoring 43%. The highest antibiotic resistance was against the cephalosporin group, followed by quinolones and macrolides. The MIC results showed that amikacin and azithromycin had a value of 4 µg/ ml, whereas levofloxacin MIC was 2 µg/ ml. FIC concentration with a borderline of ≤ 0.5 showed synergistic activity against MRSA strains with a combination of amikacin and levofloxacin.
Conclusion
An antibiotic combination therapy of amikacin and levofloxacin produces a synergistic effect against MRSA, thereby significantly increasing anti-biofilm efficacy and feasibility of preventing or delaying the formation of resistance.
1. INTRODUCTION
S. aureus presents a significant challenge in healthcare settings due to its high prevalence and predisposition for nosocomial transmission. As a leading cause of healthcare-associated infections (HAIs), S. aureus significantly contributes to patient mortality and morbidity. A study reported that in U.S. hospitals, S. aureus is among the top pathogens responsible for central-line-associated bloodstream infections (CLABSIs) [1]. MRSA has been classified as a high-priority pathogen by the World Health Organization (WHO) due to the observed emergence of these strains. According to the WHO, MRSA infections are associated with noticeably increased rates of septic shock, post-infection hospital stays, ICU duration of stay, ICU mortality, and infection-attributable mortality. According to CDC assessments, MRSA has been reported as a serious threat after considering diverse factors, such as trouble with healthcare infrastructure and the community, prevalence, and escalating trends of resistance, treatability, mortality, preventability, and transmissibility [2, 3]. Tertiary care hospitals encounter several significant challenges in their efforts to prevent MRSA infections, including overcrowding, outdated facilities, deficiencies in healthcare worker training, and the high expenses associated with infection control measures [4]. MRSA and vancomycin-resistant Staphylococcus aureus (VRSA) represent critical public health challenges in India, as recent research has shown an increasing prevalence of these pathogens. The Indian Council of Medical Research (ICMR) documented a rise in the prevalence of MRSA, escalating from 32.9% in 2017 to 38.6% in 2018. The most significant rates were recorded in North India at 52.8%, with West India following closely at 48.1%. The prevalence of VRSA in India has been reported to vary between 2% and 7.1% [5].
S. aureus has become characteristically resistant to most antibiotics, such as beta-lactams, cephalosporins, and low-level aminoglycosides. S. aureus has gained resistance to the antibiotic methicillin by possessing a resistance-causing gene, mec A, encoding Penicillin-binding protein 2a (PBP 2a) against the β-lactam drugs due to the evolution of a genetic variation subtype called Methicillin-resistant S. aureus (MRSA) [6-8]. It has been reported that the probability of in-vivo resistance to all beta-lactam antibiotics, including cephalosporins and carbapenems, to which they may appear sensitive in vitro, increases when MRSA is isolated from any infection [9]. Research has also shown that the added ability of MRSA to form structured bacterial colonies encased in an extracellular polymeric matrix or biofilm is essential to its pathogenicity and persistence. MRSA is responsible for constant and recurrent infections because these biofilms shield it from external stresses, including immune reactions and antibiotic treatments. In clinical settings, MRSA biofilms are especially hazardous since they are linked to endocarditis, chronic wounds, and infections of implanted medical devices [10]. MRSA shows antibiotic resistance through the PBP2a and PBP2c proteins, which are produced by the mec A and mec C genes, respectively. The staphylococcal chromosomal cassette mec (SCCmec) contains these genes, with mec A encoding PBP2a, an alternative penicillin-binding protein that is responsible for the high-level methicillin resistance of MRSA [11]. Efflux pumps play a crucial role in the antibiotic resistance exhibited by Staphylococcus aureus, particularly in MRSA. These transmembrane proteins function by actively transporting a range of antibiotics out of bacterial cells, which leads to a decrease in intracellular concentrations of the drugs and a subsequent reduction in their effectiveness. This mechanism not only facilitates multidrug resistance but also contributes to the virulence of bacteria and the formation of biofilms [12]. Recently, the prevalence of vancomycin-resistant Staphylococcus aureus (VRSA) has been reported to develop resistance to vancomycin via the horizontal transfer of the van A gene cluster, which is commonly sourced from vancomycin-resistant enterococci (VRE). The van A operon comprises several essential genes, namely Van H, Van A, and Van X, which play a critical role in conferring antibiotic resistance [13]. S. aureus causes severity, with reports showing its colonization in about 30% of the human population with the primary cause of bacteremia, infective endocarditis (IS), osteoarticular, pleuropulmonary, skin and soft tissue, and device-related infections [14].
To tackle the health risks linked to MRSA and VRSA, it is necessary to conduct surveillance, implement suitable infection control measures, predominantly in healthcare facilities, and ensure that life-saving medicines resistant to MRSA are readily available. The conventional protocol for determining antibiotic susceptibility involves the disc diffusion method; however, determining the minimum inhibitory concentrations (MICs) by dilution methods is a reference standard utilised to determine the limit of antimicrobial activity of the medications [15]. As the bacteria become resistant to persistent antibiotics, drug combinations are receiving more consideration [16].
Studies have reported that the successful combination of antibiotic treatments, ciprofloxacin-daptomycin [17] and daptomycin-fosfomycin [18], was the active combination against MRSA. The activities of vancomycin, tigecycline, and rifampin against MRSA were demonstrated in a rat model [19]. On the other hand, antimicrobial combinations can be antagonistic, as in the case of ciprofloxacin and levofloxacin combined with rifampin against MRSA [20]. Amikacin belongs to the family of aminoglycosides and has been widely used in the cases of critical care units [21]. Similarly, fluoroquinolones have been the best combination drug for most of the synergistic studies against MRSA [17, 20, 22, 23]. Furthermore, macrolide azithromycin has been commonly used orally for the treatment of upper respiratory tract infections [24].
To our knowledge, no such studies of combination antibiotic therapy using amikacin with levofloxacin, azithromycin with levofloxacin, and amikacin with azithromycin showing synergistic and antagonistic activity have been conducted. The present study focuses on investigating the prevalence of MRSA in a Hyderabad tertiary care facility, the antibiotic susceptibility of the S. aureus isolates obtained from various cases of infection, and determining the MICs for the standard. Further, we examine the in vitro synergy between the clinically relevant antibiotic combinations against a selection of MRSA isolates.
2. MATERIALS AND METHODS
2.1. S. aureus Strains
The present study was undertaken at the Department of Microbiology at a tertiary care hospital in Hyderabad from December 2021 to August 2023, and it was carried out with the Institutional Review Board approval with IRB no 2023/39/012 dated 22/02/2023 and obeyed the tenets of the declaration of the Helsinki.
All the clinical samples were submitted to the microbiology department from the patients attending different OPDs, and also from patients admitted in IPDs and ICUs and were processed within 2 h of the collection as per the standards.
Inclusion criteria: All S. aureus strains obtained from clinical samples were incorporated into the study.
Exclusion Criteria: Patients who were either discharged or transferred from other healthcare facilities within three days following the diagnosis of S. aureus infections, as well as those who experienced an episode of infection within a timeframe of less than three months, were excluded. Patients diagnosed with cellulitis who did not exhibit any discharge were not included in the study.
Moreover, the study included various clinical samples, including blood, pus, sputum, urine, and other bodily fluids. All samples except urine were cultured on blood agar, MacConkey agar, and nutrient agar. Cysteine lactose electrolyte deficient agar (CLED Agar) was used for urine [25]. S. aureus was identified according to standard microbiological methods, such as colony morphology, Gram stain, catalase test, coagulase, mannitol fermentation test, and DNase production [6]. ATCC 29213 was used as the reference strain for this investigation. The sample size calculation was performed as per the previously described studies [26].
2.2. Detection of MRSA Strains
2.2.1. Cefoxitin Disk Diffusion (CDD)
The suspension of all the isolates was adjusted to 0.5 McFarland to establish lawn culture on Muller Hinton Agar (MHA) plate, then subjected to a cefoxitin disc diffusion test using 30 µg disc. The plate was read after incubation at 37 °C for 18 to 24 h. Isolates showing a zone of inhibition of ≤ 21 mm with cefoxitin were considered MRSA [27].
2.2.2. Oxacillin Disc Diffusion (ODD)
All the isolates were tested by oxacillin disc diffusion using 1 μg disc after making a lawn culture of 0.5 McFarland suspension of isolates on the MHA plate. The plate was read after incubation at 37 °C for 18 to 24 h. Isolates showing a zone of inhibition of ≤ 10 mm with cefoxitin were considered MRSA.
2.2.3. Polymerase Chain Reaction (PCR)
DNA template preparation was performed using a QIAamp DNA mini kit (CAT NO: 51306) for DNA extraction. The bacteria were pelleted by centrifugation for 10 minutes at 5000 rpm. The bacterial pellet was suspended in 180 µl of lysozyme with a concentration of 20 mg/ml. The sample was incubated for at least 30 minutes at 37°C and vortexed thoroughly. The vial was loaded into the QIAcube automated instrument, which was preloaded with proteinase K, AL buffer, AW1 and AW2 wash buffers, and elution buffer to undergo the process of extraction.
2.2.4. Quality Control
Negative (ATCC 29213) and positive controls (ATCC 43300) were included in each batch of DNA extraction. DNA purity was checked by measuring its absorbance at a 260/280 ratio. PCR steps were carried out by previously published protocol [28].
For mec A gene PCR, the forward primer 5’- GTAGAAATGACTGAACGTCCGATAA and reverse primer 5’- CCAATTCCACATTGTTTCGGTCTAA were used. S. aureus ATCC 29213 was used as positive control, and E.coli ATCC 25922 was a negative control strain for this investigation.
2.3. Antimicrobial Susceptibility Pattern
The antimicrobial susceptibility pattern was determined by Kirby Bauer's disc diffusion method as per the Clinical and Laboratory Standards Institute (CLSI) guidelines. The antibiotics tested were ciprofloxacin (5 μg), levofloxacin (5 μg), amikacin (30 μg), ampicillin (10 μg), gentamicin (10 μg), co-trimoxazole (5 μg), clindamycin (2 μg), erythromycin (15 μg), azithromycin (15 μg), linezolid (30 μg), teicoplanin (30 μg), cephalexin (30 μg), cefotaxime (30 μg), cefoxitin (30 μg), oxacillin (1 μg) and vancomycin (30 μg). The zone of inhibition was checked after incubation at 37 °C for 18 to 24 h, and the results were interpreted as per the CLSI guidelines [29].
2.4. Biofilm Detection
The technique for detecting biofilms was performed using the microtiter plate assay. Microtiter plate with 96-well U bottom polystyrene titer plate (Thermofisher Scientific, India) was seeded with a 1:100 diluted ratio of 106 CFU/ ml S. aureus culture in Brain Heart Infusion (BHI) broth. The study followed the criteria set out by Stepanovic et al. [30] for the biofilm production interpretation. The formation was reported using OD values: OD <0.120 indicates a non-biofilm producer, OD range between >0.120 and < 0.240 indicates a moderate biofilm producer, and OD >0.240 indicates a strong biofilm producer. Biofilm formation against single antibiotics and combination were also tested.
2.5. Determination of Minimum Inhibitory Concentration (MIC)
The broth microdilution method was used to ascertain the MIC against amikacin, cephalexin, levofloxacin, and azithromycin. MIC was performed using CLSI guidelines and was read as the lowest concentration without visible growth [31].
Age group | Gender |
MRSA (n=84) |
p-value* (χ2 test) | Significance | |
Female | Male | ||||
0-15 | 5 | 14 | 19 (22.6 %) | 0.577 |
Not significant |
15-30 | 5 | 3 | 08 (9.5 %) | ||
30-45 | 11 | 9 | 20 (24 %) | ||
45-60 | 10 | 15 | 25 (30 %) | ||
>60 | 10 | 2 | 12 (14.2 %) | ||
Total | 41 | 43 | 84 | 0.514 (Not significant) |
2.6. Checkerboard Synergy Test
The effectiveness of different antibiotic combinations was measured using the checkerboard synergy method [23]. The average fractional inhibitory concentrations index (ΣFICs) was calculated as follows:
![]() |
Where,
![]() |
![]() |
The combination is synergistic when the ΣFIC is 0.5, indifferent when the ΣFIC is > 0.5 to <4, and antagonistic when the ΣFIC is > 4.
2.7. Time-kill Test
Based on the FIC results, the time-kill assay for single antibiotics, namely, amikacin, azithromycin, levofloxacin, and combinations of amikacin+ levofloxacin, amikacin+ azithromycin, and azithromycin+ amikacin were performed on strong biofilm-producing MRSA and compared to the control (untreated organisms) [32].
Primarily, the MRSAs were grown on MH broth medium at a concentration of 2 x 104 CFU/ ml. Following this, to the bacterial culture, antibiotics were added singly or in combination for MRSA. The suspensions were then incubated at 37oC. To estimate the remaining live bacteria using surface drop method, samples were taken at regular intervals of 0, 2, 4, 6, 8, and 12 h and diluted at a 1:10 ratio. Further samples were processed in an MH agar medium to estimate the number of MRSA bacteria. The mean numbers of viable bacteria were counted and displayed against the time of the incubation period. The experiments were performed in triplicates.
2.8. Statistics
The statistical analysis was performed using SPSS software version 20, and figures were plotted using Prism GraphPad. The Chi-square test was used to evaluate the relationship between the demographic components; a p-value of less than 0.05 was considered significant. Regression analysis was performed for assessing the relationship between resistance patterns and clinical outcomes.
Clinical samples | No. of MRSA (n=84) | Percentage (%) | p-value* (χ2 test) | Significance |
CCU | 25 | 30% | 0.99 | |
OPD | 9 | 11% | ||
PICU | 9 | 11% | ||
NICU | 8 | 9.5% | ||
ORTHO | 7 | 8.3% | ||
OT | 4 | 5% | Not significant | |
PGW | 4 | 5% | ||
GMW | 3 | 3.6% | ||
MICU | 3 | 3.6% | ||
FSW | 3 | 3.6% | ||
SICU | 1 | 1.2% | ||
Labor Ward | 1 | 1.2% | ||
COVID WARD | 1 | 1.2% |
Clinical Samples | No. of MRSA (n=84) | p-value* (χ2 test) | Significance |
Blood | 36 (43%) | 0.83 | |
Pus | 29 (35%) | Not significant | |
Urine | 9 (11%) | ||
ET secretion | 4 (4.8%) | ||
Catheter Tip | 3 (3.6%) | ||
Wound Swab | 1 (1.2%) | ||
Sputum | 1(1.2%) |
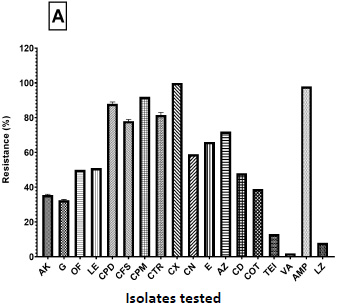
Percentage of resistance of the isolates tested against different antibiotics.
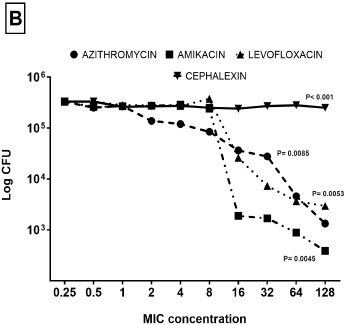
Minimum inhibitory concentrations (MIC) of azithromycin, amikacin, levofloxacin, and cephalexin antibiotics against MRSA strains. The data represents the log reduction of the CFU/ ml as derived from three independent experiments.
3. RESULTS
3.1. Demographic Characteristics
A total of 3,663 clinical samples from various sources, such as exudate, urine, blood, and body fluids, were processed over 2 years (December 2021 to August 2023). Of these, 185 isolates were identified as S. aureus, of which 84 (45%) were MRSA and 68 were MSSA. The detection of MRSA by disc diffusion was performed as recommended by the CLSI guidelines. Out of the 185 isolates, 73 were found to be MRSA by oxacillin disk diffusion (ODD), whereas 84 isolates were resistant to cefoxitin disc diffusion, and 76 isolates (93%) showed the presence of mec A gene amplification by PCR.
Table 1 shows the demographic data of the patients; out of 84 MRSA isolates, males had a higher prevalence of 51% compared to females showing 49%. Most MRSA isolated were in the age group 45-60 years with 30%, followed by 24% belonging to the age group of 30-45 years and the least of 9% between 15-30 years, as shown in Table 1.
According to our data, Table 2 shows that the MRSA prevalence was high, with 30% in critical care unit (CCU) followed by 11% in the pediatric intensive care unit (PICU) and outpatient department (OP). In comparison, 9.5% was found in the neonatal intensive care unit (NICU), and the lowest of 1% was from the surgical intensive care unit (SICU) and labor ward. The clinical history of these samples are sepsis, septic shock, and encephalopathy. Among MRSA, the highest number was seen in blood at 44%, followed by 29% in pus and urine at 12%, and the least 1% was seen in wound swabs and sputum Table 3.
3.2. Pattern of Resistance
Among a total of 84 MRSA, 45 isolates (54%) were found to be multi-drug resistant. The combined graph Fig. (1A) displays the resistance pattern of S. aureus isolates to the tested antimicrobials. The highest multi-drug resistance was seen in males (30%) compared to females (24%). The majority of isolates were sensitive to vancomycin, linezolid, and teicoplanin. CCU ward showed the highest multi-drug resistance, and prevalence was higher in blood samples (50%). The highest antibiotic resistance was against the cephalosporin group (91.5%), followed by macrolides (61%) and quinolones (51%). Among other groups of antibiotics, glycopeptides (98%), oxazolidinones (92%), and aminoglycosides (67%) were more active against MRSA isolates. A total of seventy-six (n = 76) isolates were identified as mec A positive through PCR analysis from eighty-four MRSA isolates (n = 84). The prevalence of the mecA gene was 95% (n = 76/84), while eight isolates (n = 8/84; 9.5%) did not show the mec A gene. All eight samples exhibited a sensitive pattern. The MIC method indicated that only one sample was sensitive to amikacin and azithromycin. For levofloxacin, six samples showed sensitivity, while two samples showed resistance. Both the MIC and Kirby-Bauer methods revealed complete resistance for cephalexin.
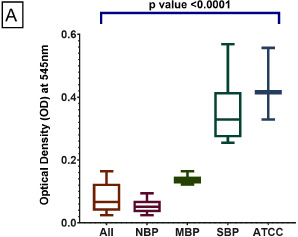
Biofilm formation by MRSA strains was assessed, classifying them into non-biofilm producers (NB), Moderate Biofilm (MB), and Strong biofilm (SB) on U bottom polystyrene titer plate surface stained with crystal violet after incubation of MRSA strains and quantitative analysis of biofilm production by measuring the optical density at 595 nm. The data represents mean ± SD values as derived from three independent experiments.
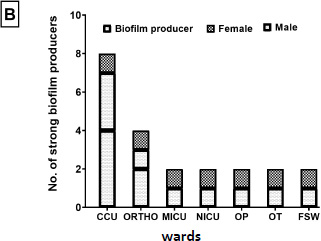
Ward-wise and gender distribution of the strong biofilm producers. The data represents mean ± SD values number of microorganisms as derived from three independent experiments.
3.3. Biofilm Detection
Out of the 84 MRSA samples analysed, 11 (13%) showed strong biofilm production, 62 (74%) were identified as non-biofilm producers, and another 11 (13%) isolates displayed moderate biofilm production (Fig. 2A). The distribution of males and females among the 22 biofilm producers was equal, with each gender representing 50%. The predominant sources of strong biofilm producers were the CCU ward patients, with males making up the majority, followed by the ORTHO ward, having equal numbers of males and females, reflecting equal gender distribution. The other wards, such as MICU, NICU, OP, OT, and FSW wards, all had a single female patient with no males (Fig. 2B). All individuals identified as biofilm producers exhibited multi-drug resistance. The effect of biofilm formation against combination antibiotics showed a significant reduction in MRSA compared to single antibiotics and control (Fig. 2C). All the strong biofilm producers showed a general resistance to the tested antibiotics amikacin, azithromycin and levofloxacin.
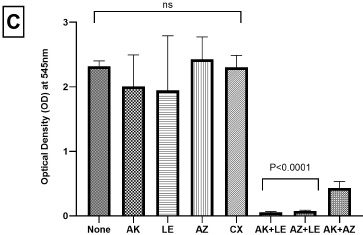
Effect on biofilm formation of MRSA treated with single antibiotics single antibiotic – amikacin (8 μg/ ml), azithromycin (32 μg/ ml), levofloxacin (8 μg/ ml) and combinations of amikacin+ levofloxacin, amikacin+ azithromycin and azithromycin+ amikacin were performed on the strong biofilm producers MRSA using MIC range and compared to the control (untreated organisms) showing inhibition of viable activity. Each data point represents an optical density at 595 nm. The data represents mean ± SD values as derived from three independent experiments.
3.4. MIC
The MIC range for amikacin was 0.05 µg/ ml to 8 µg/ ml (Fig. 1B). Thirteen samples (15.5%) proved sensitivity to amikacin, while thirty-nine samples (46.4%) showed moderate sensitivity. The MIC assay for azithromycin showed a range of 1 to 32 µg/ ml. However, sensitivity was present in twelve samples (14.3%), while intermediate sensitivity was found in fourteen samples (17%). The MIC value of levofloxacin was 1 to 32 µg/ ml, with twelve samples (14.2%) immediately responsive to levofloxacin, whereas five samples (6%) showed sensitivity to the antibiotic. A MIC of 16 µg/ ml for cephalexin was effective against just four isolates (4.8%), and the remaining isolates showed complete resistance at 128 µg/ ml.
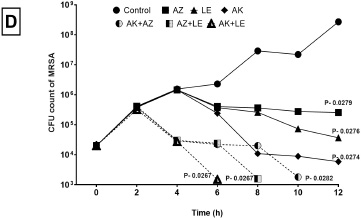
Time kill assay of MRSA treated with single antibiotics – amikacin (8 μg/ ml), azithromycin (32 μg/ ml), levofloxacin (8 μg/ ml), and combinations of amikacin+ levofloxacin, amikacin+ azithromycin and azithromycin+ amikacin were performed on the strong biofilm producers MRSA using MIC range and compared to the control (untreated organisms) showing inhibition of viable activity. The data represents the log reduction of the CFU/ ml as derived from three independent experiments.
3.5. Checkerboard Synergy Test Results
Of the tested three combinations, only two combinations, amikacin plus levofloxacin and azithromycin plus levofloxacin, showed an excellent synergistic effect in comparison to amikacin plus azithromycin showing an additive effect for 46 and an antagonistic effect for 31 isolates. Out of the 84 MRSA isolates, the mean FIC of amikacin, azithromycin, and levofloxacin combinations showed that amikacin plus levofloxacin had a practical synergistic effect in 45 isolates, an additive effect in 27, an antagonistic effect in 11, and no effect in 1 isolate.
3.7. MRSA Killing Rate
The MRSA isolates were grown with antibiotics in single and combinations further, and the viability was checked at different time intervals. Fig. 2D indicates that the combination of amikacin and levofloxacin drastically reduced the MRSA count after 4 h and was fully eliminated after further incubation hours. With the Azithromycin+ levofloxacin combination, the reduction was observed after 6h; however amikacin+ azithromycin combination showed reduction at 8 h. MRSA grown in the single antibiotic suspensions showed a reduction in the count of bacteria; however, no killing effect was observed when compared to the controls.
4. DISCUSSION
Globally, the number of cases of MDR S. aureus is increasing. Hence, diagnosing MRSA in the laboratory is vital for hospital patient management. This study aimed to determine cumulative antibiograms (Fig. 1A) as per CLSI guidelines. After analysing 185 S. aureus isolates from clinical specimens Table 2, the overall prevalence of MRSA isolates was found to be 45.4%, less than the reported data of 70.64% by Sapkota et al. [33], 52.4% by Bouchiat et al. [34], and 46.78% by Kale et al. [35]. Cefoxitin is regarded as a stand-in marker for oxacillin and is a more effective medication in detecting the mec A gene in MRSA. While comparing the gender distribution of MRSA infections, males (51%) were more affected than females (49%). Similar trends were observed by Garg et al. [36], with 69.7% male and 32.1% female; Roy et al. [37] showed similar results with higher males at 55.6% and females at 44.4%. This study also shows that more MRSA isolates were from inpatients (89%) than outpatients (11%). Similar findings were observed by Lohan et al. [38] for inpatients (75.3%) and outpatients (24.7%). In comparison to ward-wise distribution, MRSA prevalence was higher in CCUs. Patients in an ICU, especially a surgical ICU, have wounds, drains, and invasive monitoring devices that cause skin breaches, which further increases the risk of developing infections [29]. Similar results of 43% were concluded by Wattal et al. [39] and 58.3% by Qodrati et al. [40]. Among all clinical samples, the highest rate of MRSA isolation (43%) was from blood samples, followed by pus (29%). A similar observation was made by Hassoun et al. [41]. The high prevalence in blood samples can be due to prolonged hospitalization and misuse of antibiotics. Methicillin resistance was detected using the CDD and ODD methods. In our study, ODD found 87% sensitivity, and CDD showed 100% sensitivity; a similar finding was reported by Lohan et al. [38] from New Delhi. Among 84 MRSA, 45 samples (54%) were multi-drug resistant. The highest multi-drug resistance was observed in males (30%) compared to females (24%) [42]. The highest antibiotic resistance pattern was seen in cephalosporin (91.5%), followed by macrolides (61%) and quinolones (51%) by the Kirby–Bauer disc diffusion method. Similar findings were observed by Lohan et al. [38] and Dash et al. [43].
The gold standard for MRSA confirmation is detecting the mec A gene or its product, PBP 2a protein. In our research, 84 MRSA isolates were tested for the presence of the mec A gene. Among these, 76 isolates tested positive for mec A, representing 95%, while 8 isolates were negative for the mec A gene. Comparable results were reported in a hospital study, and the PCR analysis revealed that thirty-three (94%) of the 35 MRSA isolates tested positive for the mec A gene, with only two isolates (6%) negative for mec A [44]. Similar results were reported in a study in a nearby tertiary care center of Karimnagar, Hyderabad, from November 2018 to October 2019, where 48 MRSA isolates were tested for the mec A gene, and all 48 isolates were positive for the mec A using the PCR method [45]. A study characterized MRSA by phenotypic and genotypic methods and revealed that the mec A gene was identified in 9 out of 10 MRSA isolates among healthcare workers and its containment in a tertiary care hospital in South India [46].
Our research indicates that the formation of biofilms was high in multidrug-resistant strains; similar findings were shown in a previously described analysis [47]. According to the previous findings, a study reported that out of 113 MRSA samples, 38 isolates exhibited significant biofilm production, while 14 isolates demonstrated low levels of biofilm production [48]. In our study, the biofilm-producing isolates were resistant to most antibiotic tests; similar observations have been reported in most studies [49, 9]. Our study shows the prevalence of more biofilm formation by the isolates from CCU; a ward-level record study in Singapore has demonstrated similar reports [50, 51]. Since critical care patients are more prone to nosocomial infections, stricter control measures should be implemented.
Research studies have recommended the determination of MIC in cases where the organisms have an elevated level of resistance, patients are at risk, and in the case of difficult-to-access infections [37, 52]. The increased resistance to cephalexin has been of grave concern lately; a similar resistance profile of 84.6% was shown in HIV patients study from 2012-2017 [53]. The expanding resistance profile to first-generation cephalosporins- cephalexin shows the high-level use of this antibiotic for treating S. aureus infections.
The constructive interaction between antibiotics can enhance their antibacterial activity and also help to reduce the development of antibiotic resistance. A similar study was conducted with vancomycin plus rifampicin, vancomycin plus aminoglycoside, and Vancomycin plus rifampin and aminoglycoside [13]. In China, the combination of fosfomycin and vancomycin was used to check its synergistic effect. It was helpful for high-organism-burden infections in healthcare settings [54]. Synergic combinations of levofloxacin with clindamycin and levofloxacin with oxacillin have been reported to be effective by time-killing assays [22]. Another study showed the superior synergism with the ciprofloxacin-levofloxacin-rifampicin combination [20]. The findings of the checkerboard assay and time-kill trials suggest that the combination antibiotics (amikacin plus levofloxacin and azithromycin plus levofloxacin) work in synergy [20, 55]. The synergistic effect of amikacin and levofloxacin can be attributed to their complementary mechanisms of action, so that they affect bacteria at multiple levels. Amikacin binds to the 30S ribosomal subunit, inhibiting protein synthesis and causing bacterial death. Levofloxacin is a fluoroquinolone that interferes with DNA replication and repair by inhibiting DNA gyrase and topoisomerase IV. In summary, this combination enhances the antibacterial activity of bacteria because it increases the inhibition of protein synthesis of the DNA replication process and prevents bacterial proliferation in this process. Studies have shown that these combinations are very effective, especially against diseases, such as ESKAPE disease, which poses a great challenge in medical practice [56]. This study shows that these combinations could be more effective than antibiotics in treating MRSA infections. Antibiotic combinations, such as amikacin in conjunction with levofloxacin or azithromycin paired with levofloxacin, show synergistic effects.
Clinicians may consider implementing these combinations as adjunctive therapies, particularly in cases of multi-drug resistant (MDR) or complicated MRSA infections. The study enhances the need for implementing hospital-based antibiotic stewardship programs and enhances genotypic-based diagnostic capabilities for rapid identification of resistant strains [57]. Focusing on bacteriophage therapy, antimicrobial peptides/ nanoparticles, and probiotics as long-term strategies for MRSA prevention is a forward-thinking approach [58-60]. However, the limitation of this study is that this was carried out in a single healthcare facility, which may limit the generalizability of the findings to other settings. Additionally, the distinction between hospital-acquired and community-acquired MRSA was not addressed. In the susceptibility testing assay, no resistance to vancomycin was found; hence VAN gene was not isolated.
CONCLUSION
This study showed a prevalence of 45% MRSA isolates from the tested clinical samples of 3,663. MRSA prevalence was higher among male patients (51%), in critical care wards (30%), and in blood samples, which accounted for 43%. The highest levels of antibiotic resistance were seen against cephalosporins, followed by quinolones and macrolides. Minimum Inhibitory Concentration (MIC) testing revealed values of 4 µg/ ml for amikacin and azithromycin and 2 µg/ ml for levofloxacin. Fractional Inhibitory Concentration (FIC) indices ≤ 0.5 indicated synergistic activity between amikacin and levofloxacin against MRSA isolates. Therefore, this combination therapy significantly enhances anti-biofilm activity and offers promising results in preventing biofilm formation and decreasing the burden of AMR in MRSA isolates.
AUTHORS’ CONTRIBUTIONS
K.P. and N.S: contributed to designing the majority of the experiments and took part in interpreting the data and conceptualizing the study; K.P. and N.S: contributed to writing the manuscript; V.G.P. and A.K.O: Contributed to revising the manuscript; All the authors read and approved the last version of the manuscript as submitted and agreed to be accountable for all aspects of the work.
LIST OF ABBREVIATIONS
MRSA | = Methicillin-resistant S. aureus |
MIC | = Minimum inhibitory concentration |
CDD | = Cefoxitin disk diffusion |
ODD | = Oxacillin disc diffusion |
FIC | = Fractional inhibitory concentration |
HAIs | = Healthcare-associated infections |
CLABSIs | = Centralline-associated bloodstream infections |
WHO | = World Health Organization |
MHA | = Muller Hinton Agar |
PCR | = Polymerase Chain Reaction |
CLSI | = Clinical and Laboratory Standards Institute |
MDR | = Multi-Drug Resistant |
ETHICS APPROVAL AND CONSENT TO PARTICIPATE
Approval from the Department of Microbiology at a tertiary care hospital in Hyderabad, Institutional Review Board with IRB no 2023/39/012 was taken on 22/02/2023.
HUMAN AND ANIMAL RIGHTS
All procedures performed in studies involving human participants were in accordance with the ethical standards of institutional and/or research committee and with the 1975 Declaration of Helsinki, as revised in 2013.
AVAILABILITY OF DATA AND MATERIALS
The data sets used and/or analysed during this study are available from the corresponding author [N.S] upon request.
ACKNOWLEDGEMENTS
Declared none.