All published articles of this journal are available on ScienceDirect.
Reconnoitering the Association of Vaginal Microbiota Composition in the Female Reproductive Tract with Health and Disease
Abstract
Vaginal microbiota plays a crucial role in women's reproductive health, and its composition varies throughout different stages of life. In particular, pregnancy alters the vaginal microbiota, with Lactobacillus spp. being predominant in healthy pregnant women. Dysbiosis during pregnancy is linked to adverse outcomes, such as preterm birth. Menopause leads to changes in the vaginal microbiota due to declining estrogen levels, characterized by reduced Lactobacillus sp. and increased diversity of other bacteria, potentially contributing to health issues, such as vaginal dryness and increased susceptibility to infections, such as bacterial vaginosis (BV), vulvovaginal candidiasis (VVC), and preterm birth. This in turn also leads to the risk of being more susceptible to cancer, such as cervical cancer caused by human papillomavirus (HPV), breast cancer, and ovarian cancer. Non-communicable disorders, such as obesity and diabetes mellitus, also influence the vaginal microbiota and affect reproductive health outcomes. Interactions between vaginal microbiota and sexually transmitted infections (STIs) further influence disease susceptibility and progression. Understanding these dynamics is crucial for developing interventions to promote healthy vaginal microbiota and improve reproductive health in women. This comprehensive review examines the evolution of vaginal microbiota from birth through menopause and its implications for various health conditions and reproductive outcomes and to manage potential imbalances in this ecosystem.
1. INTRODUCTION
Our bodies harbor a diverse community of microorganisms, collectively known as the microbiota. These tiny residents play a crucial role in shaping our development, physiology, immunity, and even how we obtain nutrients [1]. Most of these microbes live in harmony with us, forming a mutually beneficial partnership. However, a small number pose potential threats, causing chronic infections or even life-threatening diseases [2].
One key function of the microbiota is to protect against harmful invaders. They out-compete disease-causing organisms, preventing them from colonizing our bodies. Despite their importance, the intricate details of these communities, including variations between individuals and the complex interactions within them, remain largely a mystery. However, extensive research efforts are underway to unlock the hidden aspects of microbiota and understand their true impact on our health and well-being [3].
Traditionally, research is focused primarily on the role of microbiota in disease states. However, the focus has shifted towards recognizing the fundamental role of our resident microbes in maintaining overall health. Just like the gut and mouth, the female vagina harbours a vibrant ecosystem of billions of microorganisms, including bacteria, viruses, and fungi. This ecosystem exemplifies the finely balanced partnership between humans and microbes. We provide the necessary nutrients for their growth while they help maintain a healthy vaginal environment [4].
While we know about various species present in the vaginal microbiome, their specific functions and influence on the overall community dynamics remain largely unknown. It is believed that this intricate ecosystem has evolved through co-evolution between humans and specific microbial partners, though the exact selective pressures driving this partnership are yet to be fully understood. Recognizing this, researchers are actively exploring ways to maintain a healthy vaginal microbiota (VMB) through more natural and effective solutions [5].
Well-characterized probiotic bacteria, primarily certain Lactobacillus species, hold promise in maintaining vaginal health or restoring the natural balance after antibiotic use. Studies suggest that probiotics may offer potential benefits in addressing various conditions. This review provides an overview of the vaginal microbiota, the key factors influencing their composition, and how they change throughout a woman's life. Understanding these changes and their impact on health may pave the way for developing effective strategies to maintain a healthy VMB and promote overall well-being.
2. METHODS
2.1. Research Questions
This review aimed to address the following research questions:
1. What is the composition and role of the vaginal microbiota (VMB) throughout different stages of a woman's life?
2. How does the vaginal microbiota influence general and reproductive health?
3. What is the association between vaginal dysbiosis and non-communicable and communicable diseases and cancer?
4. Which microbial and host factors regulate immune homeostasis in the vaginal environment?
2.2. Inclusion and Exclusion Criteria Inclusion Criteria
The articles were included based on the following criteria:
• Peer-reviewed original research articles and systematic reviews.
• Studies examining the composition and function of the vaginal microbiota in different age groups of women.
• Research investigating the relationship between VMB and reproductive health outcomes.
• Studies focusing on VMB alterations during diseased conditions, including pregnancy, menopause, obesity, PCOS, and cancer.
• Articles published in the English language.
• Publications from the past 10 years to ensure up-to-date relevance.
Exclusion criteria
The articles meeting the following criteria were excluded:
• Editorials, commentaries, opinion articles, and non-indexed publications.
• Studies lacking primary data or clear methodology.
• Animal studies not directly related to human VMB implications.
• Articles not available in full-text format.
• Publications not focused on the VMB or related health impacts.
2.3. Search Strategy
A structured literature search was performed using PubMed, Scopus, Web of Science, and Google Scholar databases. The search terms included combinations of keywords and MeSH terms, such as “vaginal microbiota”, “vaginal dysbiosis”, “Lactobacillus”, “reproductive health”, “immune regulation”, “pregnancy outcomes”, “non-communicable diseases”, “bacterial vaginosis”, “vaginal infections”, “cancer”, “PCOS”, “obesity”, “HIV”, and “biofilm”.
Boolean operators (AND, OR) were used to refine the search. An example of the search string is provided as follows: (“vaginal microbiota” OR “vaginal flora”) AND (“Lactobacillus” OR “dysbiosis”) AND (“pregnancy” OR “cancer” OR “PCOS” OR “immune”) AND (“health” OR “disease”).
The search was restricted to studies published between January 2014 and the present date. Reference lists of relevant studies were manually scanned to identify additional sources.
2.4. Study Selection
Study selection occurred in two phases as follows:
Phase 1: Titles and abstracts were screened by two independent reviewers based on predefined criteria.
Phase 2: Full-text articles were retrieved for studies meeting the criteria. These were reviewed independently, and disagreements were resolved through the involvement of a third reviewer.
2.5. Data Extraction
Data from the selected studies were extracted using a standardized form, including i) author(s), year, country; ii) study design and population; iii) key microbial findings (composition, dominant species, diversity, species specificity); iv) host-related factors (age, hormonal status, disease condition); v) associations with health outcomes; vi) associations with reproductive outcomes; and vii) notable conclusions and implications.
Two reviewers independently completed the extraction process, and disagreements were resolved through conversation.
2.6. Quality Assessment
Included studies were assessed for quality using appropriate tools based on study type. For observational studies, the Newcastle-Ottawa Scale was applied. Clinical trials were evaluated using the Cochrane risk-of-bias tool. Each study was scored by two reviewers, with consensus used to resolve discrepancies.
2.7. Data Synthesis
Due to the diversity of study designs and outcomes, a narrative synthesis was used. Findings were grouped by themes, such as:
• Vaginal microbiota throughout different life stages
• Role of Lactobacillus in health and disease
• VMB dysbiosis in non-communicable and communicable diseases
• VMB involvement in cancer risk
• Biofilm formation and immune interactions
3. THE VAGINAL MICROBIOTA
The VMB is a diverse microscopic ecosystem residing within the female reproductive tract. It primarily comprises bacteria, with Lactobacillus species playing a dominant role. These “friendly” bacteria produce lactic acid, creating a slightly acidic environment (pH 3.8-4.5) that discourages the growth of harmful pathogens. Other bacterial residents include Atopobium, Bifidobacteria, Gardnerella vaginalis, and Prevotella, each contributing to the VMB's delicate balance. Additionally, fungi, like Candida, and viruses may be present in smaller numbers, but their specific roles remain under investigation [6, 7].
The VMB's composition is not static. Maintaining a healthy VMB is crucial for female reproductive health. It acts as a barrier against infections, helps maintain vaginal pH, and potentially influences the immune system. Understanding these influences is crucial for maintaining women's reproductive and overall health, enabling tailored interventions to preserve a healthy vaginal microbiota and prevent complications associated with dysbiosis. As research delves deeper into the VMB, it paves the way for developing strategies to prevent and manage various women's health issues [7]. Fig. (1 shows the factors that influence the VMB composition and their contribution to women’s lives.
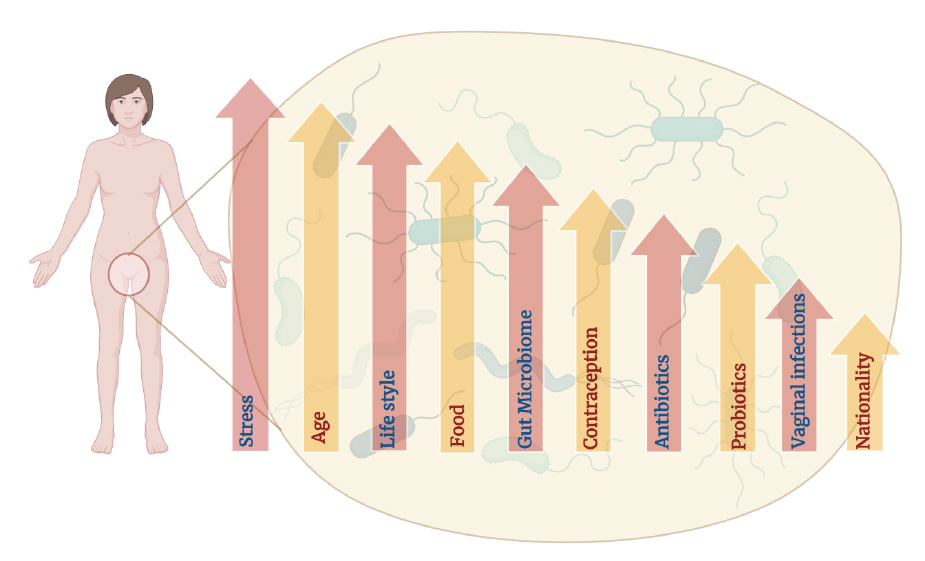
4. PHYSIOLOGICAL ADAPTATION FOR SURVIVAL OF VAGINAL MICROBIOTA
The VMB thrives in a specific microenvironment characterized by a slightly acidic pH (3.8-4.5). This acidic niche is primarily established by dominant Lactobacillus species, which ferment vaginal secretions to produce lactic acid. This not only creates an inhospitable environment for harmful pathogens, but also contributes to optimal pH maintenance for the VMB [8]. Beyond pH, the VMB requires essential nutrients for survival. The vaginal epithelium sheds cells, providing a constant source of maintenance. Additionally, cervical mucus and other secretions further contribute to the nutritional landscape, ensuring the VMB's resource availability. Interestingly, the vaginal environment is microaerobic, characterized by low oxygen concentration. This oxygen-limited state favours the growth of anaerobic bacteria, like Lactobacillus, allowing them to dominate the VMB and maintain a healthy equilibrium [9].
The VMB interact closely with the vaginal mucosa, a protective layer lining the vagina. This mucosa plays a critical role in immune defense by secreting antimicrobial molecules and housing immune cells that actively eliminate potential pathogens, thereby safeguarding the delicate balance of the VMB [10].
5. MICROBIAL LANDSCAPE OF THE VAGINAL ENVIRONMENT
While Lactobacillus species, particularly the L. delbruekii group (L. crispatus, L. iners, L. jensenii, and L. gasseri), are the dominant players, constituting up to 90% of the VMB in healthy individuals, their specific roles and interactions within the VMB are an area of ongoing research [11]. The presence of fungi, like Candida sp., and viruses has also been documented within the VMB, although their precise functions and significance remain under investigation. Understanding their role in the ecosystem may provide valuable insights into maintaining a healthy VMB.
5.1. Influence of Vaginal Microbiota on Age
Women undergo too many physiological challenges and changes compared to men. These changes are largely driven by hormonal fluctuations. Hormonal changes affect women and men differently due to variations in hormone levels, reproductive anatomy, and biological processes. In every stage of life from birth, including puberty, reproductive age, and menopause, the composition of VMB is mainly impacted [6]. In the early stages of life, the mother's vaginal microbiota predominantly colonizes the exterior and interior surfaces of a child delivered vaginally. Additional colonizers are acquired from the mother’s skin and oral microbiota. Recent years have shown that breast milk contains distinct microbiota that are passed to nursing children and are primarily dominated by Lactobacilli [12]. The early infancy vaginal microbiota is made up of several anaerobes, including Diphtheroids, coagulase-negative Staphylococci, Corynebacterium spp., Mycoplasma spp., and E. coli [9]. There exists a dearth of research on VMB of infants and young females (1 to 12 years) before puberty.
Puberty marks the beginning of reproductive capability in girls, characterized by the development of secondary sexual characteristics, such as breast development and menstruation. Menstruation, a monthly cycle regulated by hormones, involves the shedding of the uterine lining if fertilization does not occur. The vaginal microbiota before puberty were previously believed to consist of comparatively stable populations of aerobic, anaerobic, and enteric bacteria [13]. After menarche, the vaginal microbiota of healthy adults are characterized by a high concentration of homo-fermentative lactic acid bacteria, which produce lactate and other organic acids, leading to the vaginal environment becoming more acidic [14]. The clusters of vaginal microbiota in adolescent girls, at both pre and post-menarche, are characterized by high proportions of different Lactobacillus spp. Another cluster has the proportions of S. agalactiae, S. anginosus, Prevotella, and Anaerococcus [15]. Some adolescent girls rarely harbour the population of A. vaginae, G. vaginalis, and genital mycoplasmas [16-18].
The reproductive years involve menstruation, pregnancy, and motherhood for many women. Challenges during this stage may include fertility issues, pregnancy-related complications, balancing work and family responsibilities, and societal expectations regarding motherhood and childcare. The VMB are greatly impacted by fluctuations in the amounts of hormones used to regulate the menstrual cycle, such as progesterone (P) and estradiol (E2). Sexual hormone levels could impact the thickness of the epithelial barrier, the frequency of immune cells, and vaginal microorganisms that are resident in the female genital canal, all of which are defensive barriers [19, 20]. During the reproductive years between 20–30 and 31–43 years of age, the VMB of women includes a diversity of Paraprevotella, A. vaginae, Ochrobactrum, L. manihotivorans, Varibaculum, Granulicatella, E. coli, P. intermedia, Shewanella, Gardnerella, and Enterococcus [21].
Menopause, which typically occurs between the ages of 45 and 55, marks the end of the reproductive years. It is characterized by reduced ovarian function, which lowers progesterone and estrogen output [22]. Additionally, menopausal women are at increased risk for conditions, such as osteoporosis and heart disease, due to the decline in estrogen levels. For women aged between 55-75 years, it is believed that Lactobacilli are either absent or present in low concentrations, glycogen is either completely absent or drastically decreased, and there is a risk of vaginal epithelium atrophy [22-24]. There is an inclination towards the colonization of E. coli, Enterococci, and other gram-negative organisms [25, 26]. Fig. (2) depicts the diversity of the VMB, and their abundance varies throughout a woman’s life from birth to death.
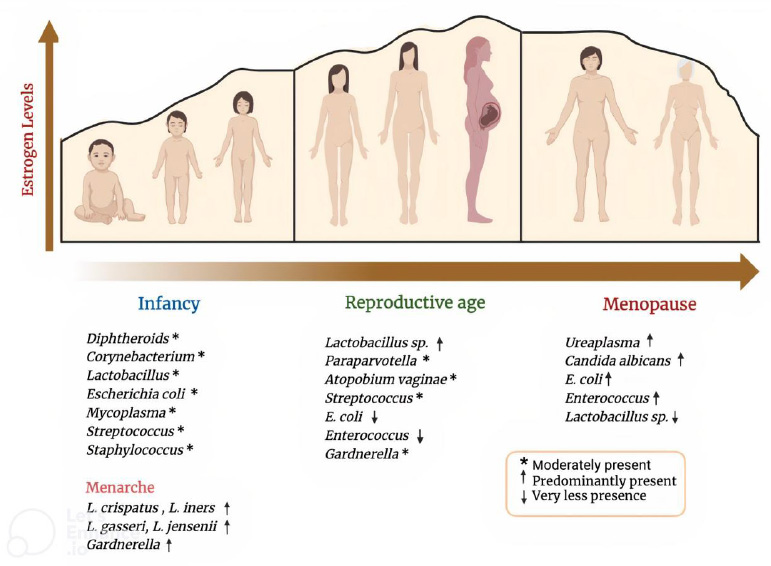
6. IMPACT OF VAGINAL MICROBIOTA DURING PREGNANCY
Pregnancy brings about profound physiological changes as the body adapts to support the developing fetus, including hormonal shifts, weight gain, and changes in organ function. The VMB of pregnant individuals can undergo fluctuations and transition between different community state types (CSTs) [27]. Studies show that most of the pregnant women have healthy vaginal microbiota with many Lactobacilli counts and greater stability throughout the gestational period. A significantly lower population of pregnant women have vaginal dysbiosis. A pioneering longitudinal study employing DNA sequencing techniques in both non-pregnant and pregnant women revealed that pregnant individuals who delivered at term rarely exhibited high-diversity microbial communities. Women who give birth at term tend to have vaginal microbiota predominantly composed of L. crispatus. Furthermore, pregnant women have been found to have more Lactobacilli than non-pregnant women [27-29].
A study found that during the last half of pregnancy, there is a gradual decrease in Lactobacillus abundance in the vaginal microbiota, while bacteria, like Moraxella, Streptococcus, Staphylococcus, Enterococcus, Corynebacterium, and Gemella, increase. This suggests that changes in the vaginal microbiota during pregnancy occur progressively rather than suddenly at labor onset or membrane rupture [30].
The vaginal microbiota directly influence pregnancy outcomes, like term or pretem delivery (PTD) [31]. A significant proportion of asymptomatic women of reproductive age are colonized by glucose-consuming species other than Lactobacilli, such as Streptococcus spp., Atopobium vaginae, Prevotella spp [32], L. gasseri, L. johnsonii, L. iners, Ralstonia solanacearum, Bifidobacterium longum, B. breve, L. crispatus, and L. acidophilus. Colonization by certain Lactobacillus species within the vaginal microbiota, like L. crispatus and L. acidophilus, may be associated with a reduced risk of preterm delivery (PTD), whereas vaginal community state types (CSTs) not dominated by Lactobacillus species may be linked to an increased risk of PTD [33].
7. ROLE OF LACTOBACILLI IN HEALTHY VAGINA OF PREGNANT WOMEN
Although numerous studies have investigated the relationship between vaginal microbiota and pregnancy outcomes, a few have provided detailed explanations of the mechanisms underlying adverse events. However, the protective properties of Lactobacillus species against pathogen colonization are widely acknowledged. Lactobacilli utilize glycogen breakdown products in the vagina to generate lactic acid, thereby creating an acidic pH that inhibits the growth of various bacteria. Additionally, they up-regulate autophagy, facilitating the clearance of intracellular pathogens from vaginal epithelial cells [34]. To further establish their dominance over other bacteria, Lactobacilli also generate bacteriocins [35, 36]. L. gasseri, L. crispatus, and L. jensenii can produce both the D and L-isomers of lactic acid, whereas L. iners has a genome reduction gene that is necessary for producing D-lactic acid. Regarding reproductive tract health, research indicates that the D-isomer of lactic acid can suppress the production of matrix metalloproteinase-8 (MMP-8). This enzyme breaks down the cervical plug, which acts as a barrier preventing bacterial entry into the upper genital tract [34-36].
8. VAGINAL MICROBIOTA OF PRE- AND POST-MENOPAUSAL WOMEN
Women, upon reaching the end of their reproductive ages, typically enter menopause, which spans the final third of their lives. This stage is defined by alterations in sex hormone levels and the onset of various symptoms related to aging. Ultimately, this menopause condition induces variations in the composition of the VMB, mainly composed of Candida albicans, G. vaginalis, U. urealyticum, and Prevotella sp., together with a progressive decrease in species of Lactobacillus [9].
A study reported VMB dysbiosis in pre-, peri-, and postmenopausal women to reveal novel community assemblages associated with vulvovaginal atrophy in postmenopausal women. Perimenopausal women tend to be dominated by L. iners. Notably, among Lactobacilli, L. iners stands out as some strains may exhibit pathogenic characteristics. It is currently understood that certain strains of L. iners may not offer the same level of protection in maintaining a stable vaginal environment. L. crispatus and L. iners have been found to be more prevalent in premenopausal women [24, 25, 37].
A comprehensive study of vaginal microbiota in pre-menopausal women aged 39-42 years and post-menopausal women aged 58-62 years showed species richness to be significantly higher in the pre-menopausal group compared to post-menopausal women. Analyzing the vaginal microbiota composition at the family level exhibited a significant decrease in Lactobacillaceae post-menopause (24.7% compared to 65.9%), while several other families, including Peptoniphilaceae, Veillonellaceae, Actinomycetaceae, Coriobacteriaceae, Pseudomonadaceae, Morganellaceae, Enterobacteriaceae, and Prevotellaceae, showed noteworthy increases in post-menopausal women. At the genus level, Lactobacillus exhibited substantial reductions post-menopause (23.7% compared to 63.2%), whereas Prevotella, unclassified Lactobacillaceae, Finegoldia, Proteus, Pseudomonas, Escherichia, and Atopobium showed significant increases after menopause. Notably, the Lactobacillus genus decreased from 63.2% pre-menopause to 23.7% post-menopause [38].
9. VMB COMPOSITION DURING THE INCIDENCE OF NON-COMMUNICABLE DISEASES (NCDs)
The composition of a healthy vaginal microbiota (VMB) is significantly influenced by the prevalence of NCDs in women, including diabetes mellitus, obesity, PCOS, heart disease, asthma, and allergies. The VMB alterations during NCDs have not been studied well. Inadequate research has been performed on the effects of vaginal microbiome dysbiosis on allergies, asthma, and heart disorders.
9.1. VMB and Diabetes Mellitus
Women with diabetes mellitus are more likely to be affected by yeast infections, like candidiasis, since their vaginal environment is rich in sugar. Candida vaginitis is a tremendously common condition, affecting approximately three of four women worldwide at least once in their lifespan. It might promote the occurrence and recurrence of vaginal candidiasis in women having diabetes mellitus. It can be challenging for healthcare professionals to offer evidence-based guidance on how to break subsequent bouts of candidiasis because a significant portion of the pathophysiology and epidemiology are still unclear [3].
During pregnancy, due to increasing weight and hormonal changes, women tend to get gestational diabetes mellitus. The occurrence of this metabolic disease is quite common in the third trimester of pregnancy. The high ratio of Prevotella/Aerococcus in the vaginal environment is also correlated to high blood glucose levels [39-41]. In the type 2 diabetes mellitus (T2DM) cohort in a study, Lactobacillus did not decline with the number of years following menopause. These patterns could indicate that T2DM impedes the reduction of Lactobacillus or that lowering estrogen levels in elderly diabetic patients has a limited effect on controlling Lactobacillus count [40].
The presence of gestational diabetes mellitus (GDM) has been linked to specific strains of Lactobacillus in the vaginal microbiota. Studies have identified L. listeri, L. amylovorus, and L. fructivorans as being associated with GDM. Conversely, the constitutional ratio of vaginal L. iners has been found to be decreased in individuals with GDM [41].
9.2. VMB and Obesity
The prevalence of obesity has escalated globally, with projections indicating that by 2025, approximately one in five women will be classified as obese, and one in ten will be categorized as morbidly obese [42]. Vaginal microbiome (VMB) dysbiosis and obesity are risk factors for negative reproductive and oncological health outcomes in women [43]. Numerous studies have indicated obesity to have a harmful effect on women's health, including early menarche in young obese girls, anovulation, adult pregnancy loss, and irregular menstrual cycles. Additionally, a recent study found that obese women have a distinct ratio of several vaginal Lactobacillus species, indicating that non-vaginal health issues, like obesity, can have an impact on the vaginal microbiota [44]. A study analyzed the association between vaginal microbiota and obesity and confirmed the adverse impacts of obesity on the vagina, showing the significantly increased diversity and abundance of Sneathia as well as Prevotella [45].
The correlation between estrogen levels and obesity is complex and not fully understood. In postmenopausal women, the process of aromatization of androgens in peripheral fat becomes the primary mechanism for estrogen production, replacing ovarian function [46]. In a case-control study conducted in the UK involving 67 obese women and 42 control subjects, it was discovered that obese individuals were less inclined to possess a VMB dominated by Lactobacillus. Additionally, they exhibited reduced levels of L. crispatus and a higher relative abundance of Prevotella timonesis, Dialister sp., and A. vaginalis, compared to women of healthy weight [43]. In a cross-sectional study comprising nearly 6000 participants in the USA, it was revealed that overweight and obese women exhibited a greater prevalence of bacterial vaginosis (BV) in comparison to thin women [47].
9.3. VMB and Polycystic Ovarian Syndrome (PCOS)
PCOS is one of the most common endocrine illnesses; the symptoms typically include irregular menstruation, hirsutism, and infertility. The composition of lower genital tract microbiota could greatly impact menstruation in PCOS women. A vaginal tract with reduced Lactobacillus sp. of PCOS patients could greatly impact follicular stimulating hormone (FSH) concentrations. The depletion of this symbiotic microbe provides the scope for the growth of other possible pathogens, including Gardnerella, Prevotella, Veillonellaceae, Chlamydia, Streptococcus, and Dialister species. Reduced levels of Lactobacillus spp. in the female reproductive tract have been linked to the beginning of vaginosis, premature labor, stillbirth, infertility, repeated implantation failure, abortion, and many other unfavorable pregnancy outcomes [48].
In a case-control study conducted between PCOS patients and controls, mycoplasma showed dominance in patient samples compared to controls [49]. M. hominis appeared to be elevated in patients with PCOS. Similarly, another case-control study reported Prevotella to be higher in patients and that Actinomyces could be a potential biomarker to identify PCOS. Streptococcus may affect the endocrine system in female reproductive organs, which could impact PCOS pathophysiology [50].
10. VAGINAL MICROBIOTA AND CANCER
10.1. VMB and Cervical Cancer
The composition of the VMB also has an impact on the rate of human papillomavirus (HPV) clearance and, consequently, the risk of developing a malignant transformation linked to HPV. More specifically, VMBs having higher concentrations of Lactobacillus or L. iners have been linked to faster HPV remission, whereas microbiota containing higher concentrations of Atopobium and smaller quantities of Lactobacillus have been linked to delayed HPV clearance. It is interesting to note that L. gasseri positively correlated with both a higher rate of remission and a higher level of HPV infection [24, 51]. According to certain theories, Chlamydia trachomatis infections in the vagina may also change an individual's microbiota, making them more vulnerable to HPV infection [52].
Co-infection with microbes may increase the risk of HPV-related carcinogenesis by altering HPV transcription and replication. Moreover, co-infection worsens inflammation and harms the epithelium, which serves as a route for HPV infection [53]. Changes in the HPV status are linked to alterations in the VMB, which in turn leads to the risk of cervical cancer. Both past and present HPV infections have been linked to higher levels of pathogenic Gardnerella species, while Atopobium was significantly detected only in samples that tested positive for HPV. Nevertheless, Prevotella bivia, Trachoma chlamydia, Streptococcus agalactiae, N. gonorrhoeae, Mycoplasma/Ureaplasma spp., M. hominis, Candida albicans, Diallisteria, and Prevotella timonensis have all been found to be associated with HPV infection prevalence [54].
In a study analyzing the impact of vaginal microbiota on cervical cancer patients, researchers observed slight disturbances in women with cervical cancer compared to those with less pronounced dysbiosis. Additionally, cases of aerobic vaginitis caused by Streptococcus sp. were noted, and patients without normal VMB exhibited diversified vaginal infections [19, 54]. The presence of another member, like Sneathia spp., can be a microbiological marker for cervical neoplasm, HPV infection, and increased human immunodeficiency virus (HIV) acquisition [55].
10.2. VMB and Ovarian Cancer
The role of VMB in pathogenesis has emerged as a cutting-edge area of study. Lack of Lactobacillus dominance and the prevalence of ovarian cancer are directly proportional. This has established the first link between vaginal dysbiosis and ovarian cancer. Bacteria from the vaginal microbiota, including Clostridium and Lachnospiraceae, have been observed to correlate either positively or negatively with the development of ovarian tumors [56, 57].
Research examining the impact of M. genitalium, C. trachomatis, or Neisseria gonorrhoeae on ovarian cancer has indicated a potential connection between these microbes and the development of the disease. The results of the research have found an association between double ovarian cancer risk with Pgp3 and the antibodies against Chlamydia plasmid-encoded protein, which is the gold standard in determining Chlamydia infection [21]. Regardless of menopause, the altered VMB typically resemble those in healthy postmenopausal subjects. This has been evident in a greater diversity of microbes and an increase in Corynebacterium and Propionibacterium, which have even been observed in the initial stages of ovarian clear cell cancer and mucinous ovarian cancer [58]. This suggests that the development of ovarian cancer could be linked to aberrant VMB with reduced protective activity [57].
10.3. VMB and Breast Cancer
Breast cancer is the most prevalent type of cancer in women and the leading cause of cancer-related death in women worldwide. It accounts for roughly 15% of all cancer-related fatalities [59]. Vaginal infections (e.g., C. trachomatis or N. gonorrhoeae) increase the risk of developing breast cancer [60]. Studies have demonstrated that fluctuations in the ratio of Firmicutes to Bacteroidetes bacteria can result in higher levels of deconjugated estrogen. Elevated circulating free estrogen, in turn, enhances binding to estrogen receptors (α and β), contributing to the development or progression of estrogen-dependent conditions, such as endometrial and breast cancers, as well as endometriosis [61].
In a study, the researchers investigated the VMB of breast cancer patients. They observed a notable decrease in the total count of Lactobacillus spp., yet no statistically significant variations were found in microorganism numbers among patients with different breast cancer subtypes. Comparative analysis revealed obligate anaerobic bacteria, particularly Peptostreptococcus spp., to be predominant in the vaginal microbiota of patients with luminal A and luminal B subtypes. In contrast, Staphylococcus spp., facultative anaerobic organisms, were more prevalent in cases of Her2/Neu+ and triple-negative subtypes associated with unfavourable outcomes [62].
11. VAGINAL MICROBIOTA IN INFECTIONS AND COMMUNICABLE DISEASES
During communicable diseases, disruptions in the balance of vaginal microbiota can occur, potentially increasing susceptibility to infections. Changes in the composition and diversity of vaginal microbiota may affect the host's immune response and create an environment conducive to the growth of pathogens. Few studies have been done on the VMB since the onset of communicable diseases. There are not many studies that have concentrated on the same aspect. The gonorrhoea-causing bacterium, N. gonorrhoeae, is constantly becoming resistant to ceftriaxone and azithromycin, two antibiotic treatments, which makes recovery more difficult. According to an in vitro study, Lactobacillus can release biosurfactants, produce bacteriocins, create an acidic environment, co-aggregate with gonococci, and decrease gonococci's adhesion to epithelial cells. All these actions can drastically limit the survival of Neisseria gonococcus [59-63].
11.1. Vaginal Microbiota and HIV Infection
HIV infection is the most predominant sexually transmitted infection (STI). Humoral immune mediators have been investigated as a potential mechanism to explain the correlation between HIV acquisition and vaginal dysbiosis, including pro-inflammatory chemokines and cytokines. Increased levels of a pro-inflammatory cytokine, such as interleukin-1β (IL-1β), linked to tissue damage and toll-like receptor (TLR) signaling, have been repeatedly linked to altered vaginal microbiota (Nugent score 4-10). Vaginal discharge contains an HIV-inducing factor (HIF), which has been linked to BV since the 1990s. This element enhances HIV-1 replication in T cells and monocytes by activating AP-1 and NF-κB. Significant evidence has been found linking the vaginal bacterial species M. hominis, commonly associated with BV, to HIF. By compromising the cervicovaginal mucus barrier and other innate immune responses against HIV, BV may further increase the risk of HIV infection by facilitating HIV's entry into the genital epithelium. Additionally, it has been demonstrated that the synthesis of metabolites, such as short-chain fatty acids, by bacteria associated with BV is connected to the stimulation of pro-inflammatory gene pathways in vaginal epithelial cells as well as the increased production of pro-inflammatory cytokines [64].
12. VAGINAL MICROBIOTA AND INFECTIONS
12.1. Bacterial Vaginitis (BV)
BV predominantly affects women, especially in reproductive age. The importance of Lactobacillus in the vaginal environment is largely discussed. One more milestone is lacking Lactobacillus abundance, leading to the onset of bacterial vaginosis, which is defined as the presence of several anaerobic species, such as G. vaginalis, Anaerococcus, A. vaginae, Bacteroides, U. urealyticum, M. hominis, bacterial vaginosis-associated bacteria type 1 (BVAB1), BVAB2, BVAB3, Leptotrichia, Mobiluncus, Peptostreptococcus, Peptoniphilus, Prevotella, and Sneathia [65]. The strains recently isolated from the vagina of a woman with BV are as follows: Olegusella massiliensis strain KHD7T, Ezakiella massiliensis strain Marseille P2951T, and Corynebacterium fournierii strain Marseille P2948T [66-68]. BV raises the possibility of contracting STDs, including T. vaginalis, N. gonorrhoeae, C. trachomatis, HPV, herpes simplex virus type 2 (HSV-2), and HIV. Moreover, BV increases the chance of developing pelvic inflammatory disease, chorioamnionitis, endometritis, and amniotic fluid infections [69].
12.1.1. Gardnerella: A Potential Pathogen Causing BV
The most prevalent microbe found in the vaginal samples of BV patients is Gardnerella [70-72]. But when it comes to figuring out if BV is positive or negative, G. vaginalis has shown great sensitivity and low specificity. There are several virulence factors linked to G. vaginalis's pathogenic potential; the most well-studied ones are sialidase and vaginolysin. G. vaginalis contributes to the breakdown of vaginal mucus barriers by using sialidase to hydrolyze sialic acid residue from mucus sialoglycans in the vagina and subsequently catabolizing free carbohydrates. A few Gardnerella species are noteworthy for having negative sialidase activity. These include G. vaginalis, G. leopoldii, and a specific subgroup of G. swidsinskii [72].
Gardnerella sp. is not alone in producing sialidase and glycosulphates; some of the other species of Prevotella and Bacteroids also produce the same. This leads to the dysbiotic state of the vaginal environment and results in inflammation. A study looked at the longitudinal changes in immune mediators and VMB in African women with and without BV over a period of eight weeks. The result of the study showed a rise in several pro-inflammatory markers, which can lead to inflammation and subsequent health damage. Furthermore, a recent study has demonstrated that recurrent BV might cause localized inflammation [73]. These investigations collectively imply that G. vaginalis is not the exclusive cause of BV.
12.1.2. Role of Other Microbes in BV
The mechanism of the degradation of the protective layer of the vaginal environment has been proposed based on the research examining the three different bacterial species, such as G. vaginalis, P. bivia, and A. vaginae, which describes that proteolysis by G. vaginalis promotes the growth of P. bivia. P. bivia produces an ammonia product, which promotes the growth of G. vaginalis leading to its development into a biofilm. The sialidase-producing capacity of these two strains affects the mucin layer of the vaginal epithelium. After the loss of the protective mucous layer, the adhesion of other BV-associated strains may take place, like Atopobium vaginae. Finally, the polymicrobial biofilm may form and worsen women's vaginal environment [73].
12.2. Vulvovaginal Candidiasis (VVC)
Vulvovaginal candidiasis affects almost 75% of women at least once in their lifetime, while approximately 5-10% of women with primary infection of VVC may develop recurrent VVC more than four times annually [74, 75]. Like BV, VVC pathogenesis consists of three stages: adhesion and invasion of epithelial cells, creation of a film, and secretion of virulence factors. Predominantly, mucosal infections are caused by C. albicans. Non-albicans Candida (NAC) species also cause VVC, including C. dubliniensis, C. glabrata, C. tropicalis, and C. parapsilosis [76, 77]. Even though VVC is not fatal, untreated VVC negatively impacts a person's quality of life in terms of their mental and physical well-being, social life, romantic relationships, and employment [74].
C. albicans is a commensal of a healthy vaginal environment. When a favorable condition occurs, it differentiates from yeast to hyphae and exhibits its pathogenesis [75]. Some reasonable theories regarding the transition of Candida albicans from a simple colonizer to a pathogen include vaginal dysbiosis, the expression of virulence factors (such as hyphal and biofilm formation), and the generation of proteolytic enzymes [such as secreted aspartyl proteases (SAPs)], which leads to the development of vaginal immune toxicity [76].
VVC may become more severe when it occurs alongside the activity of G. vaginalis and L. iners. A reduction of Lactobacillus population can give Candida the advantage to invade the epithelial cells of the vagina. When vaginal epithelial cells were breached, C. albicans pseudohyphae and hyphae activated the NLRP3 inflammasome receptors on the epithelial cells through a cascade, which ultimately resulted in acute vaginal inflammation [77]. Among all studies on vaginal microbiota and mycobiota, C. albicans remains the most widely recognized cause of VVC [78]. As previously mentioned, the temporary or ongoing interactions between the microbiota and mycobiota may have a role in the development of VVC in women. Determining the potential for microbial intervention and investigating these interactions are essential steps toward treating and preventing VVC in females.
13. BIOFILM FORMATION IN THE HUMAN VAGINA AND ITS ROLE IN VAGINAL DYSBIOSIS
A biofilm is defined as a microbially derived community where cells attach to a substratum, interface, or each other, embedded in a matrix of extracellular polymeric substances (EPS) they produce, exhibiting altered phenotypes concerning growth rate and gene transcription. The formation and development of a biofilm are influenced by multiple factors, including bacterial strain, surface properties, and environmental parameters, such as pH, nutrient concentration, and temperature [78].
Microorganisms within biofilms behave differently from free-floating (planktonic) microorganisms. Bacteria in biofilms exhibit increased tolerance to conventional antibiotic treatments and can more easily evade the host's immune system [79]. Biofilm formation by probiotic bacteria, such as Lactobacillus spp., is considered beneficial because it promotes colonization and prolonged presence in the host's mucosa, preventing colonization by pathogenic bacteria. It has been demonstrated that the EPS produced by some biofilm-forming Lactobacillus strains can inhibit the formation of biofilms by certain pathogenic bacteria. Indeed, adhesion and biofilm formation are strain-specific properties that contribute to the persistence of Lactobacilli in the human vagina.
However, biofilm formation has been studied more extensively in the context of BV. The formation of a polymicrobial biofilm is thought to play a key role in the etiology of BV, regardless of the proposed etiological model. As discussed, Gardnerella vaginalis is a crucial bacteria responsible for BV in many women’s vagina, playing a central role in the early adhesion stage and providing a scaffold for other microorganisms in the mature biofilm [73].
Bacterial adhesion to vaginal epithelial cells may be mediated by interactions among cell appendages (pili, fimbriae, or flagella), carbohydrates, and cell surface adhesins. G. vaginalis harbors genes encoding type I, II, and IV fimbriae/pili, as well as a biofilm-associated protein (BAP) family gene (bapL). L. iners and Peptoniphilus spp. are thought to assist G. vaginalis during the initial adhesion process. Indeed, Castro et al. showed that L. iners enhanced the adherence of G. vaginalis to epithelial cells rather than inhibiting the bacteria [79].
After adhesion and before biofilm maturation, G. vaginalis develops microcolonies and induces different symbiotic relationships. A. vaginae, Fusobacterium nucleatum, and Mobiluncus spp. may coaggregate with G. vaginalis as secondary/tertiary colonizing species, though the mechanism of coaggregation is not yet known. Moreover, uropathogenic bacteria, like Escherichia coli and Enterococcus faecalis, can co-aggregate with G. vaginalis, enhancing its growth [80]. The possible candidate bridge species between early and late colonizers may be F. nucleatum, which is well-known as the bridge species between early and late colonizers in oral biofilms, but can also reside in the vagina (in both BV and non-BV cases). Alternatively, numerous other candidate bacteria may coaggregate as late colonizers, as these bacteria have shown a synergistic interaction in a dual-species biofilm model; these include Actinomyces neuii, Bacillus firmus, Brevibacterium ravenspurgense, Corynebacterium spp., E. faecalis, E. coli, Nosocomiicoccus ampullae, Prevotella bivia, Propionibacterium acnes, Staphylococcus spp., Streptococcus spp., and G. vaginalis [73].
After successful microcolony formation/coaggregation, G. vaginalis may release extracellular DNA (eDNA) that stimulates EPS matrix production [77]. eDNA is thought to originate from lysed cells and provide structural integrity and stability to several biofilms. The release of eDNA is maximal during the early exponential growth phase of G. vaginalis, indicating its active role in biofilm formation. In addition to eDNA, the pathogenic strains of G. vaginalis (317 and 594) may encode glycosyltransferases (GT) (family I, II, and IV) that seem to be involved in EPS production. The fully matured in vivo BV biofilm appears like 'brickwork', a highly organized structure without spaces between bacterial cells.
The exact dispersal mechanism of polymicrobial BV biofilm is not known. However, it can be hypothesized that both active and passive dispersions occur during BV. Active dispersion may occur when the vaginal environment becomes favorable during menstruation, as the vaginal pH is increased by menstrual blood (pH 7.32). In contrast, passive dispersion (erosion and sloughing) may be the result of biofilm exposure to the shear forces induced by sialidase, glycosulfatase, glycosidase, proteinase, collagenase, and fibrinolysins, or the menstrual flow [78-81].
14. ROLE OF VMB IN FERTILITY
Anatomically, the vaginal environment is the most accessible site for sample collection in metagenomic studies; most of the evidence comes from this evaluation. All the conducted research proof of concept is to identify the patients with an aberrant VMB and emphasize the variations in the success of assisted reproductive treatments (RT) or pregnancies. Notably, infertile patients have asymptomatic BV associated with Candida, Enterococcus, and E. coli, and there is less Lactobacillus species. Several studies have been conducted on patients with a history of implantation failure, having an abundance of A. vaginae, L. jensenii, G. vaginalis, U. parvum, U. urealyticum, as well as Candida sp., showing elevated levels compared to normal fertile women. They have been found to be less likely to get a successful clinical pregnancy [82].
Furthermore, two distinct G. vaginalis profiles were revealed by this method; however, only one of these was associated with a low pregnancy rate, and only 6% of the women having prior conditions became pregnant following an embryo transfer [48]. Interestingly, a higher prevalence of L. crispus (above 60%) was found to be associated with a worse outcome from artificial RT. As a result, generalizations about the potential benefits of Lactobacilli for fertility should be avoided, as various species of the latter can have different effects on artificial RT outcomes [83, 84].
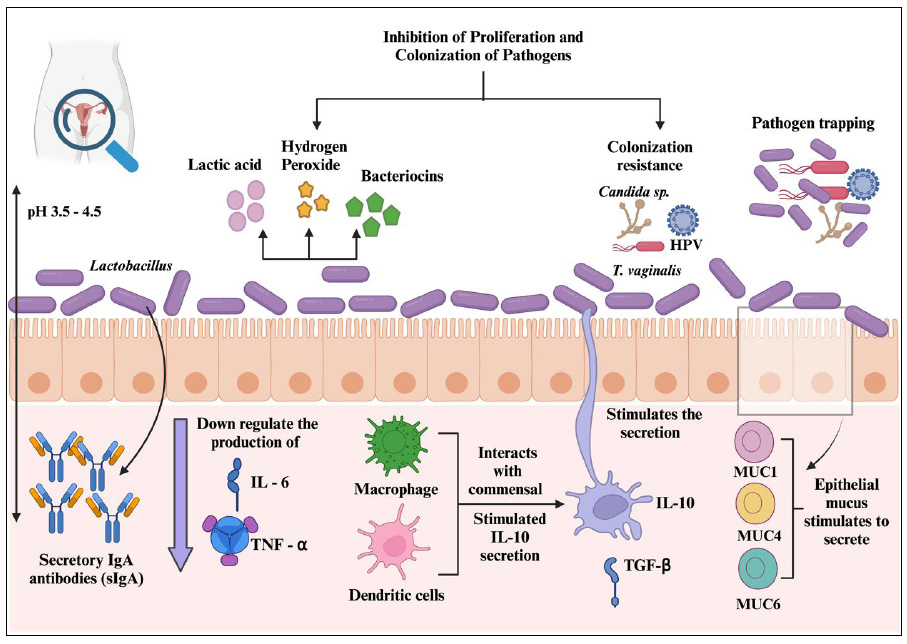
One fascinating study provided more insight into the impact of sex on the vaginal tract microbiota of infertile couples. The research methodology relied on PCR analysis of self-collected vaginal samples obtained after sexual activity as well as semen samples obtained during the partner's subsequent menstrual cycle. Although U. parvum was detected in half of the male partners, it was more common in women whose spouses had inflammatory prostatitis, accounting for 59% of the cases. The authors observed that after sexual activity, an average of four and two new species arrived and vanished in women, respectively. These changes were less common and more noticeable when normal vaginal microbiota was present [85].
15. IMMUNE HOMEOSTASIS WITHIN THE FEMALE REPRODUCTIVE TRACT MICROBIOTA
Immune homeostasis within the female reproductive tract is a critical process that ensures the delicate balance between immune defense against pathogens and tolerance toward commensal microbiota. The vaginal microbiota plays a pivotal role in maintaining immune homeostasis within the lower reproductive tract. Lactobacillus species, particularly L. crispatus, L. jensenii, and L. iners, are predominant in the vaginal microbiome and contribute to immune regulation by producing lactic acid, hydrogen peroxide, and bacteriocins. These antimicrobial compounds help sustain an acidic vaginal environment (pH 3.5–4.5), which inhibits the growth of pathogenic bacteria and reduces inflammation [86]. Lactic acid not only suppresses the proliferation of harmful microbes, but also modulates host immune responses by influencing the production of pro-inflammatory cytokines and promoting the differentiation of regulatory T cells. Studies have shown that a Lactobacillus-dominant microbiota is associated with lower levels of inflammatory cytokines, such as interleukin-6 (IL-6) and tumor necrosis factor-alpha (TNF-α), thereby preventing chronic inflammation and maintaining a stable mucosal barrier (Fig. 3) [87].
The immune system of the female reproductive tract recognizes microbial-associated molecular patterns (MAMPs) through pattern recognition receptors (PRRs), such as toll-like receptors (TLRs), NOD-like receptors (NLRs), and RIG-I-like receptors (RLRs). These receptors are expressed by epithelial cells, macrophages, and dendritic cells, allowing for the detection of both commensal and pathogenic microbes [88]. The activation of TLR2 by commensal Lactobacilli typically leads to an anti-inflammatory response, characterized by the secretion of IL-10 and transforming growth factor-beta (TGF-β), which contribute to immune tolerance and prevent unnecessary inflammation. In contrast, recognition of pathogens, such as Gardnerella vaginalis, by TLR4 induces a pro-inflammatory response, leading to increased production of IL-8 and TNF-α, which contribute to vaginal dysbiosis and bacterial vaginosis (BV) [86, 87].
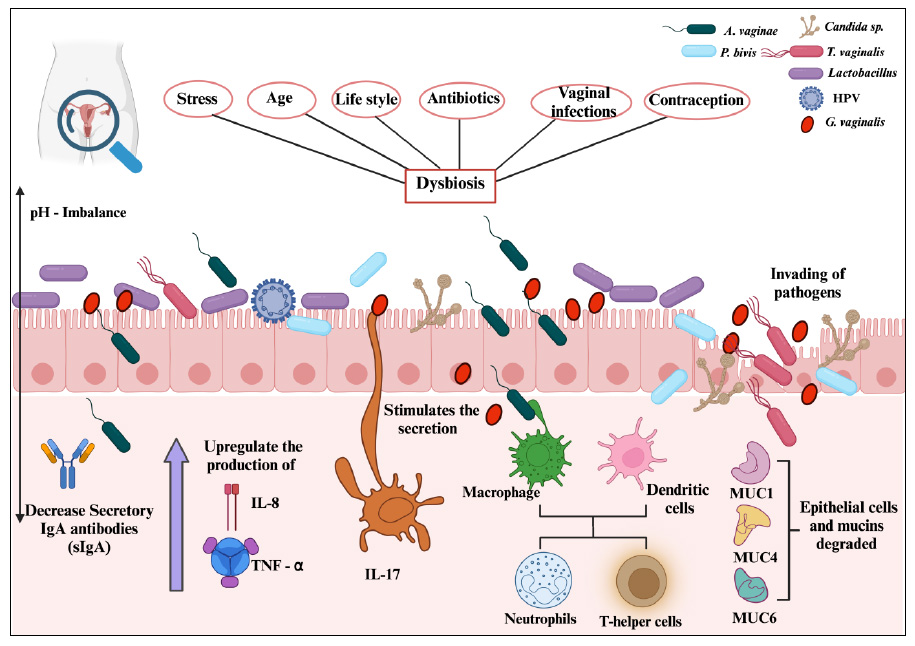
Secretory immunoglobulin A (sIgA) is another crucial component of immune homeostasis in the female reproductive tract. It serves as a first line of defense by binding to microbial antigens and preventing their adhesion to epithelial surfaces. Lactobacillus species have been shown to promote sIgA production, reinforcing mucosal immunity and reducing the risk of pathogen colonization. However, when vaginal dysbiosis occurs, characterized by a decline in Lactobacillus populations and an overgrowth of anaerobic bacteria, such as Atopobium vaginae and Prevotella bivia, sIgA levels may be diminished, weakening immune protection and increasing susceptibility to infections (Fig. 4) [88].
The epithelial barrier of the female reproductive tract is essential for immune defense and microbial regulation. The epithelial cells produce mucus, antimicrobial peptides (AMPs), and cytokines that create a protective barrier against invading pathogens. Mucins, such as MUC1, MUC4, and MUC16, prevent microbial adhesion, while AMPs, like human beta-defensins (hBDs) and cathelicidins (LL-37), exhibit direct antimicrobial activity. Lactobacillus species have been found to enhance the production of these AMPs, reinforcing immune defenses while maintaining an anti-inflammatory environment. However, disruptions to the epithelial barrier due to infections, hormonal changes, or microbial imbalances can compromise immune homeostasis and lead to conditions, such as pelvic inflammatory disease (PID) and sexually transmitted infections (STIs) [86, 89].
Dendritic cells and macrophages in the female reproductive tract play significant roles in antigen presentation and immune modulation. Under normal conditions, dendritic cells interact with commensal microbes and promote the differentiation of tolerogenic immune responses through IL-10 production. This process prevents excessive inflammation while maintaining surveillance against potential pathogens. However, in cases of dysbiosis, dendritic cells and macrophages become activated by pathogenic bacteria, leading to the recruitment of neutrophils and T-helper 17 (Th17) cells, which produce pro-inflammatory cytokines, such as IL-17 and IL-22. These cytokines can contribute to chronic inflammation, epithelial damage, and increased susceptibility to secondary infections. Regulatory T cells (Tregs) help counterbalance this response by secreting IL-10 and TGF-β, suppressing excessive immune activation, and promoting immune tolerance [87-90].
Hormonal regulation significantly influences immune homeostasis in the female reproductive tract. Estrogen has been shown to support Lactobacillus-dominated microbiota by increasing glycogen availability, which serves as a nutrient source for Lactobacilli. Estrogen also enhances epithelial barrier function by upregulating mucin and AMP expression. Conversely, low estrogen levels, such as those seen in menopause, can lead to reduced Lactobacillus abundance, increased vaginal pH, and heightened susceptibility to infections [88]. Similarly, fluctuations in progesterone levels during the menstrual cycle can influence immune responses, with higher progesterone levels generally being associated with increased immune tolerance to prevent excessive inflammation.
Disruptions in immune homeostasis within the female reproductive tract can lead to several reproductive health complications. Bacterial vaginosis, characterized by a shift from Lactobacillus-dominated microbiota to a polymicrobial biofilm with Gardnerella vaginalis, Atopobium vaginae, and other anaerobic bacteria, is associated with increased levels of pro-inflammatory cytokines, reduced sIgA, and an impaired epithelial barrier. This inflammatory state not only predisposes individuals to recurrent infections, but also increases the risk of adverse pregnancy outcomes, including preterm birth and miscarriage [89, 90].
CONCLUSION
The VMB is a critical determinant of female reproductive health, influencing immune homeostasis, fertility, and disease susceptibility. A balanced VMB, primarily dominated by Lactobacillus species, contributes to a stable vaginal environment, protecting against infections and maintaining overall reproductive health. However, disruptions in microbial composition, known as vaginal dysbiosis, have been linked to various reproductive and systemic conditions.
Dysbiosis plays a significant role in BV and VVC, where pathogenic biofilms facilitate persistent infections. Additionally, the composition of the vaginal microbiota influences susceptibility to sexually transmitted infections, including HIV. Hormonal changes, particularly during menopause, significantly alter the vaginal microbiome, leading to increased microbial diversity and heightened risks of infections and inflammatory conditions. The interplay between VMB and non-communicable diseases, such as obesity and PCOS, further underscores its systemic health implications.
Recent research has also revealed the potential association between vaginal microbiota composition and cancer risk. Disruptions in VMB have been linked to cervical cancer through interactions with HPV, while emerging evidence suggests potential roles in ovarian and breast cancer development. Furthermore, microbial imbalances may contribute to fertility challenges, including recurrent implantation failure and pregnancy complications, highlighting the need for targeted interventions.
The advancements in microbiome research emphasize the therapeutic potential of probiotics, prebiotics, and Lactobacillus-derived surface-active molecules (SAMs) in restoring microbial balance and preventing vaginal infections. Future studies should focus on biofilm disruption strategies and personalized microbiota-based therapies to improve reproductive health outcomes. Understanding the intricate relationship between vaginal microbiota and female health can prove to be essential in developing novel, targeted interventions to optimize fertility, reduce infection risks, and mitigate disease progression.
AUTHORS’ CONTRIBUTION
The authors confirm their contribution to the paper as follows: conceptualization, data curation, and original draft preparation: GV, SK; validation: JB; review and editing: PN. All authors have reviewed the results and approved the final version of the manuscript.
ACKNOWLEDGEMENTS
The figures were created using Biorender.com.