All published articles of this journal are available on ScienceDirect.
Dihydro-Cucurbitacin-E; a Secondary Metabolite of Curvularia lunata's Biotransformation as a Promising cPLA-2 and sPLA-2 Inhibitor in B[a]P-Induced Lung Toxicity
Abstract
Background
Lung cancer is the most common cause of mortality, and its prevalence is rising quickly, making it a major global health concern. Numerous studies have indicated that benzo(a)pyrene [B[a]P] in cigarette smoke is the main cause of lung toxicity.
Objective
The study's goal was to apply Curvularia lunata NRRL 2178 in biotransformation cucurbitacin-E-glucoside to dihydro-cucurbitacin-E (DHCE). We characterized the isolated DHCE using 1H-NMR and 13C-NMR spectra. We extended our study to evaluate the cancer activity of DHCE against A-549 cells in vitro, as well as its lung protective activity against B[a]P-induced lung toxicity.
Methods
We incubated Curvularia lunata NRRL 2178 with cucurbitacin-E-glucoside DHCE for 14 days. We isolated and characterized the obtained metabolite, DHCE, using 1H-NMR and 13C-NMR techniques. We also evaluated the IC50 of the isolated DHCE against A-549 cells. On the other hand, we conducted in vivo studies to assess its lung protective effect against B[a]P-induced toxicity in mice.
Results
The results of the 1H-NMR and 13C-NMR experiments showed that the metabolite, DHCE, was found because it lacked two trans-olefinic protons (23 and 24) and the hydrogen atoms of the glucose moiety in the cucurbitacin-E-glucoside skeleton structure. The IC50 value of DHCE against A-549 cells is 38.87 µg/mL, respectively. The LD50 of DHCE was 930 mg/kg b.w. Giving DHCE (18.6 and 46.5 mg/kg b.w.) orally to mice that had been given B[a]P (20 mg/kg b.w.) every day for 30 days made their plasma total cholesterol (TC), triglycerides (TG), and high density lipoprotein-c (HDL-C) levels much better, as well as their lung reduced glutathione (GSH), catalase (CAT), glutathione peroxidase (GPx), and malondialdehyde (MDA) levels, compared to mice that had been given B[a]P. On the other hand, oral administration of DHCE enhanced plasma interleukin-6 (IL-6) and nuclear factor (NF)-κB, as well as matrix metalloproteinases-2 (MMP-2) and MMP-12, in the lung of treated mice. On the other hand, administering DHCE to lung mice treated with B[a]P reduced the activity of crucial genes linked to lung inflammation, specifically cytosolic (cPLA2) and Secretory Phospholipase A2 (sPLA2). Furthermore, DHCE nearly normalized these effects in lung histoarchitecture.
Conclusion
The obtained biochemical, molecular biology, and histological results proved the lung protective activity of Curvularia lunata metabolite (DHCE) against B[a]P-induced lung toxicity in mice.
1. INTRODUCTION
Lung cancer causes an alarming number of global fatalities [1]. The International Agency for Research on Cancer's (IARC) GLOBOCAN 2020 predicts that lung cancer will cause the deaths of approximately 1.8 million individuals (18%) in 2020 [2]. Benzo[a]pyrene (B[a]P) is an aromatic polycyclic hydrocarbon (PAH) that accounts for 20 to 70% of total tobacco smoke [3]. Incomplete organic material combustion generates benzo[a]pyrene through ingestion and inhalation. Activated cytochrome P450 sequentially transforms B[a]P) into the carcinogenic epoxide benzo[a]pyrene after metabolic production [4].
World Health Organization (WHO) standards predict that chemoprevention, an alternative method, will impede the carcinogenesis process through the use of chemo- prevention substances [5-12]. Chemoprevention is a plausible pharmacological preventive method that involves the use of one or more bioactive compounds, either synthetic or naturally occurring [12-25]. Biotransformation is the most common chemical reaction that changes the biological activity of target compounds [26]. When the glucose moiety is removed from cucurbitacin-E-glucose, CE, being less polar than the glycosylated form, may have improved bioavailability [27]. This structural change affects its solubility, cellular uptake, and interactions with target proteins. CE can more easily cross cell membranes and reach its intracellular targets. Moreover, reduction the double bond between C23 and C24 in CE may affect the obtained overall conformation, stability, and binding affinity to relevant cellular targets [28, 29]. These changes could influence its ability to modulate signaling pathways involved in cancer progression.
Several fungi, including Curvularia lunata NRRL 2178, have been selected for our study to be applied in the hydroxylation reaction of steroids [29], whereas oxidation of the hydroxyl group to the corresponding keto function [30] is not a common biotransformation. Reports indicate that C. lunata breaks down the side chains of ecdysteroids, producing poststerone and rubrosterone [31].
Our study aimed to bio-transform cucurbitacin E glucoside by C. lunata NRRL 2178, evaluate the anticancer activity of the isolated dihydro-cucurbitacin (DHCE) metabolite against A-549 cells in vitro, and estimate its lung protective activity of, against B[a]P-induced lung toxicity in mice.
2. MATERIALS AND METHODS
All the chemicals used in this study were obtained from HiMedia Laboratories Pvt. Ltd. (Mumbai, India), Sigma Aldrich Chemical Co. All the chemicals used in this study were of analytical grade. Cucurbitacin-E-glucoside (98%) was obtained from Sigma-Aldrich (St. Louis, MO, USA). A549 cells are an epithelial cell line that was sourced from American Type Cell Culture (ATCC, USA, CAT: CRM-CCL-185), derived from human lung carcinoma.
The cells were maintained in Dulbecco’s modified Eagle’s medium (DMEM, Gibco, Gaithersburg, MD, USA) containing 10% (v/v) fetal bovine serum (FBS) and 5% horse serum, supplemented with penicillin/streptomycin (all from Gibco). We obtained the culture of Curvularia lunata NRRL 2178 from the Microbial Inoculants Center at Ain Shams University in Egypt and cultivated it using the conventional two-stage fermentation methodology [9].
HPLC System: Hewlett Packard 1100; Degasser: G1322A; Quaternary Pump: G1311; Auto Sampler: G1313A; Column Thermostat: G1316A; Variable Wavelength Detector: G1314A; Column: ODS C18, 25 cm x 4.6 mm, 5 μm; Mobile Phase: Methanol; Flow Rate: 0.5 ml/min.; Detection Wavelength: 275 nm (determined using a UV-Visible Shimadzu spectrophotometer).
An electrothermal apparatus determined the melting point, and an uncorrected Jasco DIP-370 digital polarimeter recorded it. IR spectra recorded in KBr using the PerkinElmer FT-IR Spectrum BX spectro- photometer. 1H and 13C NMR were measured at 400 and 100 MHz, respectively, using a Bruker AVANCE 400 spectrometer. The unaffected solvent signal at µ 8.71 for the 1H-NMR spectrum and µ 149.90 for the 13C-NMR spectrum was used as a reference for the pyridine-d5 spectrum.
2.1. Biotransformation of Cucurbitacin-E-Glucoside
Several fungi, including Curvularia lunata NRRL 2178, have been selected for our study to be applied in the hydroxylation reaction of steroids. Potato dextrose agar was used to preserve the stock culture of C. lunata NRRL 2178. C. lunata, recently cultivated on agar, was transferred into 250-mL Erlenmeyer flasks containing 100 mL of liquid medium composed of 0.1% peptone, 0.1% yeast extract, 0.1% beef extract, and 0.5% glucose [28]. Each flask was cultivated at room temperature for 72 hours, then added a solution of cucurbitacin-E-glucoside (10 mg diluted in 100 mL DMSO and 5 mL Tween 80) and extended the incubation for 14 to 17 days. Culture control consisted of a fermentation blank in which C. lunata was grown under identical conditions but without a cucurbitacin- E-glucoside substrate. Cucurbitacin-E-glucoside (28×10 mg) was fed to C. lunata in Erlenmeyer flasks (28×250 ml), each containing 100 ml of the same medium outlined above. After 14 days, the culture was filtered, and the mycelium was washed with nBuOH. Subject the crude products (450 mg) to column chromatography. Use a solvent system of dichloromethane (CH2Cl2) and methanol (MeOH) in a 92:8 ratio for elution. Isolate CE and DHCE with a yield of 281 mg and 170 mg, respectively.
The broth was extracted with n-BuOH, washed with H2O, and the solvent evaporated under reduced pressure. The crude products (450 mg) were subjected to column chromatography eluting with CH2Cl2-MeOH (92:8) to give CE (281 mg, 62.44%, TR 15.45) and DHCE (170 mg, 37.77%, TR 27.32).
2.2. Determination of DHCE Cytotoxicity against A549 Cells
To determine the cytotoxic effect of DHCE, the methyl thiazolyl tetrazolium (MTT) cell viability assay was done using 96-well plates. A549 cells were seeded at 1×104 cells/well. After 24 hours, or until a confluent monolayer was achieved, cells were treated with DHCE at different concentrations (0, 0.8, 1.6, 3.125, 6.250, 12.5, 25, 50, and 100 µg/mL). Cell viability was measured after 72 hours of treatment by removing the medium, adding 28 µL of a 2 mg/mL solution of MTT stain, and incubating the cells for 3 hours at 37 °C. After removing the MTT solution, the crystals remaining in the wells were solubilized by the addition of 130 µL of DMSO 2.5%, followed by 37 °C in a CO2 incubator (Cypress Diagnostics, Belgium) at 5% CO2 for 15 min with shaking [32]. The absorbency was determined on a microplate reader (BioTek, USA) at 492 nm, and the assay was performed in triplicate. The inhibition rate of cell growth (the percentage of cytotoxicity) was calculated using the following equation:
Cytotoxicity = [A-B/A] x 100%
Where A is the optical density of the control and B is the optical density of the samples.
2.3. Determine the LD50 of DHCE
Initial trials were conducted on cohorts of four mice. Various dosages of DHCE were administered to determine the lethal dose range, resulting in 0% and 100% mortality in animals. We measured the LD50 by administering DHCE orally at dosages ranging from 300 to 1800 mg/kg body weight. We counted the rate of animal death in separate groups during the 4-day observation period. With the increase in dose, the death rate also gradually rose. The LD50 was determined by applying the Abal et al. formula as follows [33]:
![]() |
Dm = The largest that kill all animals.
∑ = The sum of (z x d).
Z = Mean of dead animals between 2 successive groups.
d = the constant factor between two successive doses.
n = nember of animals in each group.
2.4. Experimental Animals
The study was conducted following the setting established by the Faculty of Applied Health Science Technology, October 6 University, Egypt. 30 male albino mice weighing 35 ± 2.5 grams were acquired from the National Cancer Institute at Cairo University. Animals were housed in individual cages at a temperature of 22 ± 2 °C and a relative humidity of 60%. Each animal was provided with a regular diet of ad libitum during the experimental period.
2.5. Experimental Setup
This experiment was performed to examine the lung protective activity of DHCE against b[a]p-induced lung toxicity. For 30 days, the following were treatments for 6 groups of animals, each consisting of 6 mice (Table 1).
On the 31st day, blood was collected and centrifuged, and plasma was used fresh for estimation of plasma TC, TG, and HDL-c using commercially available kits (Asan and Youngdong Pharmaceutical Co., Korea) [36, 37]. Plasma IL-6 and NF-Κb were measured using ELISA kits from RayBiotech, Inc., USA, Quest Diagnostics Nichols Institute, San Juan Capistrano, California, and R&D Systems Inc., Minneapolis, MN, USA, respectively.
Groups | Treatment Description | Treatment Description |
---|---|---|
I | Normal control | 3 mL of distilled water orally for 30 days |
II | B[a]P (20 mg/kg b.w.) (Model group) |
was given B[a]P orally in propylene glycol in a single daily dose for 30 days [34]. |
III | B[a]P + DHCE (18.6 mg/kg.b.w.) |
was treated with DHCE (18.6 mg/kg.b.w.) suspended in propylene glycol orally for 30 days. |
IV | B[a]P + DHCE (46.5 mg/kg.b.w.) |
was treated with DHCE (46.5 mg/kg.b.w.) suspended in propylene glycol orally for 30 days |
V | B[a]P + Tamoxifen (2 mg/kg.b.w) | was treated with Tamoxifen (2 mg/kg.b.w) orally suspended in propylene glycol in a single weekly dose for 30 days [35]. |
Gene | Primer’s Sequence |
---|---|
cPLA-2 | F:5′- CCTGATATGGAGAAAGATTGCC -3′ R:5′- AGGGAAACAGAGCAACG-AGA -3′ |
sPLA-2 | F:5′-GACGACAGGAAAGGAAGCC-3′ R:5′- TCCCTCTGCAGTGTTTATTGG-3′ |
GAPDH (a housekeeping gene) |
F:5′- AACTTTGGCATTGTGGAAGG-3′ R:5′- ACACATTGGGGGTAGGAACA -3 |
Lungs were dissected out, and then lung samples were homogenized in a glass homogenizer (Universal Lab. Aid MPW-309, mechanika precyzyjna, Poland). The first portion was homogenized with ice-cold saline to create a 25% w/v homogenate. We produced three separate aliquots of the homogenate. The ELISA method was used to assess lung MMP-2 and MMP-12 (Abnova, Taipei City, Taiwan) in the first aliquot. The second aliquot was deproteinized with 12% (ice-cold) trichloroacetic acid and centrifuged at 1000 ×g, and the supernatant produced was used to quantify the amount of malondialdehyde (MDA) (MyBioscience, USA) [38]. The cytosolic portion of the lung was isolated by centrifuging the third portion of the homogenate at 10500 ×g for 15 minutes at 4 °C in a cooling ultracentrifuge. We assessed the levels of GPx, CAT, and GSH using the ZeptoMetrix diagnostic kit technique.
2.6. qRT-PCR Assays
Total RNA was collected from the second part of lung specimens using real-time quantitative PCR, and sections (10–15 mg) of recovered RNA were submitted to real-time PCR analysis using Sepasol-RNA1Super according to the manufacturer’s instructions. RT-PCR was performed with 50ng of RNA template per reaction using the SensiFAST™ SYBR® Hi-ROX One-Step Kit (Meridian Bioscience Inc./Bioline; Memphis, TN, USA) in a 25μL reaction volume containing 70nM of specific primers in the Applied Biosystems 7500 Fast Real-Time PCR System (Foster City, CA, USA). The conditions of the reaction were pre-incubation at 50 oC in 2 minutes, followed by 10 min by 40 cycles of 95 oC in 15 s and 60 oC in 1 minute in 1 minute, respectively. The sequences of the primers are listed in Table 2.
2.7. Histological Assessment
The third section of lung specimens was dried in graded alcohol and embedded in paraffin after being fixed in a 10% neutral formalin solution. The fine slices (5μm thickness) were stained with hematoxylin & eosin (H&E) and mounted on glass slides for light microscopic inspection using Bancroft and Steven's approach [39, 40].
2.8. Statistical Analysis
All data was statistically assessed using the SPSS/20 program. The data were presented as mean ± SD (n = 6) and analyzed by one-way analysis of variance (ANOVA). P values less than 0.05 were considered statistically significant.
2.9. Ethical Declaration
The experiment received approval from the Ethics Committee of the Faculty of Applied Health Science and Technology (reference number 20221025). The animal research techniques were conducted according to the guidelines specified in the ninth edition of the Guide for the Care and Use of Laboratory Animals issued by the National Academy of Sciences, National Academies Press, Washington, D.C.
3. RESULTS
In the present study, cucurbitacin-E-glucoside was biotransformed by C. lunata (NRRL 2178). The isolated metabolites CE and DHCE were obtained as pale-yellow amorphous powders.
CE, the main metabolite, was isolated in 281 mg, yielding 62.44%. by CH3OH-CHCl3 as a crystallizing solvent, it showed MP 232 oC, 1H-NMR, and 13C-NMR spectral data as follows. 1H NMR (DMSO-d6, 400 MHz): δ 0.926 (1H, s, H-18), 1.11 (3H, s, 19-Me), 1.202 (2 x 1H, s, H-26 and 27), 1.99 (2H, d, H-7), 2.127 (1H, overlapping signal, H-17), 2.176 (1H, s, H21), 2.28 (1H, obscured signal, H-23), 2.499 (1H, d, H-9), 2.5 (1H, d, J = 13.6 Hz, H-1), 4.759 (2 x 1H, s, H-28 and 29), 7.3-7.4 (d, 2H, CH=CH, J= 15.7, two trans-olefinic protons H 23 and 24) and absent of hydrogen atoms of glucose moiety.
13C NMR (CD3OD, 100 MHz): δ 14.033 (C-15), 14.3 (C-32), 21.76 (C-20), 22.215 (C-4), 28.815 (C-5), 29.315 (C-6), 29.740 (C7), 31.027 (C-24), 31.413 (C-25), 38.221 (C-23), 38.947 (C-10), 39.504 (C-8), 39.782 (C-13), 40.016 (C-14), 41.78 (C-9), 42.34 (C-12), 62.347 (C-17), 69.45 (C-16), 105.33 (C-31), 120.914 (C-3), 127.857 (C-2), 129.380 (C-1), 174.915 (C-11), 183.45 (C-22), and absent of carbon atoms of glucose moiety.
DHCE, the second metabolite, was produced in (170 mg, 37.77%) yield as a pale-yellow amorphous powder with m.p. of 148-149 oC. 1H- NMR (DMSO-d6, 400 MHz): δ 0.84 (1H, s, H-18), 2.257 (1H, s, H-19), 1.22 (2× 1H, s, H-26&27), 2.08 (2H, d, H-7), 2.172 (1H, overlapping signal, H17), 2.393 (3H, s, 21-Me), 2.7 (1H, obscured signal, H-23), 3.336 (1H, d, H-9), 5.3 (1H, d, J = 13.6 Hz, H-1), 4.11 & 4.22 (2×1H, s, H-28&29) and absent of glucose moiety and olefinic protons H 23 and 24. 13C-NMR (CD3OD, 100 MHz): δ 24.336 (C-15), 18.53 (C-32), 24.336 (C-20), 25.036 (C-4), 28.971 (C-5), 29.638 (C-6), 30.643 (C7), 38.5 (C-24), 38.916 (C-25), 39.752 (C-23), 40.195 (C-10), 42.933 (C-8), 48.705 (C-13), 61.736 (C-14), 68.759 (C-9), 71.305 (C-12), 78.334 (C-17), 79.852 (C-16), 109.64 (C-31), 120.625 (C-3), 124.644 (C-2), 126.743 (C-1), 152.848 (C-11), 172.761 (C-22), and absent of carbon atoms of glucose moiety.
3.1. IC50 of DHCE
Mammalian cell lines: A-549 (human Lung carcinoma) cells were used as tumor cell lines. The cytotoxicity results of DHCE are shown in Table 3 and Figs. (1-2). IC50 value of DHCE against A-549 cells recorded 38.87 µg/mL.
3.2. LD50 of DHCE in Mice
The results given in Table 4 show that oral administration of DHCE in doses of 300, 600, 900, 1200, 1500, and 1500 mg/k.g.b.w. resulted in mortalities of 1, 3, 5, 8, 9 and 10, respectively. The dose of DHCE that killed half of the mice (LD50) was 930 mg/k.g b.w.
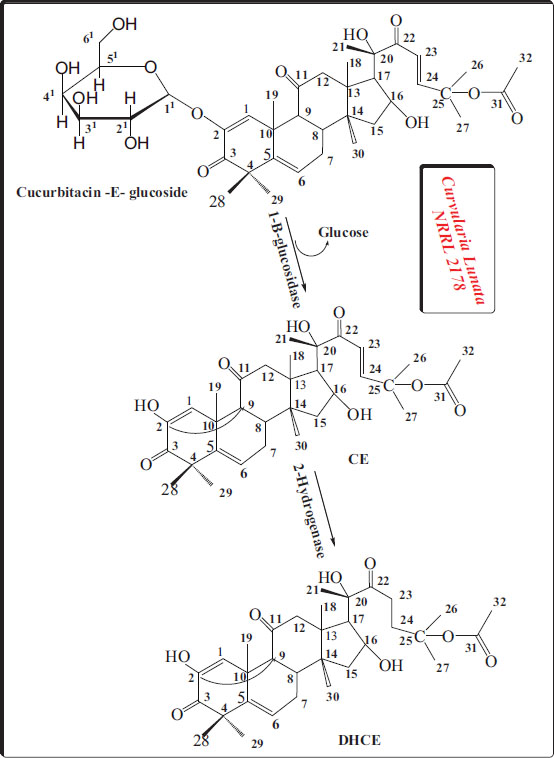
Proposed pathway for the formation of cucurbitacin-E-β-D-glucoside metabolites by Curvularia Lunata NRRL 2178.
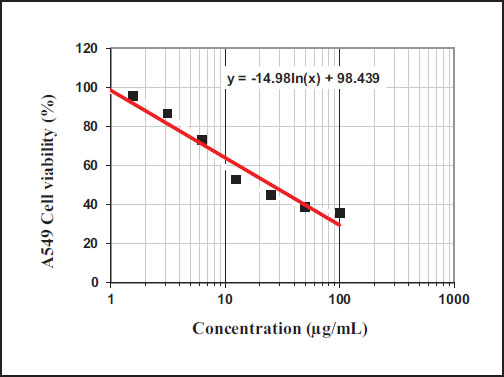
IC50 value of dihydro-cucurbitacin (DHCE) against A549 cells.
DHCE conc. (µg/ml) | Mean of Viability % | The Mean of Inhibitory % | S.D. (±) |
---|---|---|---|
100 | 35.70 | 64.30 | 2.15 |
50 | 38.90 | 61.1 | 1.65 |
25 | 44.76 | 55.24 | 1.52 |
12.5 | 52.87 | 47.13 | 1.08 |
6.25 | 72.90 | 27.1 | 0.52 |
3.125 | 86.63 | 13.37 | 1.14 |
1.56 | 95.50 | 4.50 | 0.74 |
0.8 | 98.72 | 1.28 | 0.63 |
0 | 100 | 0 | 0 |
IC50 | 38.87 ± 2.52 µg/ml |
Group Number | Dose (mg/kg) |
No. of Animals/group | No. of Dead Animals | (Z) | (d) | (Z.d) |
---|---|---|---|---|---|---|
1 | 300 | 10 | 1 | 2.0 | 300 | 600 |
2 | 600 | 10 | 3 | 4.00 | 300 | 1200 |
3 | 900 | 10 | 5 | 6.50 | 300 | 1950 |
4 | 1200 | 10 | 8 | 8.50 | 300 | 2250 |
5 | 1500 | 10 | 9 | 9.5 | 300 | 2700 |
6 | 1800 | 10 | 10 | 0 | 00 | 8700 |
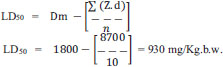
3.3. Effect of DHCE on TC, TG, and HDL-C in the Plasma of Treated Mice
Administration of B[a]P to normal mice showed a significant increase in plasma TC, TG, IL-6 and NF-kB levels by 35.65, 68.05, 39.31, and 227.15%, respectively, as well as a significant decrease (p< 0.05) in plasma HDL-C by 42.81% when compared to normal mice (Table 5). However, compared to B[a]P-exposed mice, DHCE (18.6 mg/kg b.w.) treatment resulted in a considerable decrease in plasma TC, TG, IL-6 and NF-kB by 21.80, 35.52, 12.76, and 42.28%, respectively, as well as a significant increase in plasma HDL-c by 126.25% (p< 0.05). Furthermore, the treatment of mice with DHCE (46.5 mg/kg b.w.) showed a significant depletion in plasma TC, TG, IL-6 and NF-kB by 24.60, 35.20, 24.52, and 62.18%, respectively, as well as a significant increase in plasma HDL-c of 57.49% in comparison to B[a]P-treated mice (p< 0.05). However, treatment of mice with tamoxifen showed a significant decrease in plasma TC, TG and IL-6 by 15.51, 16.05, and 19.84%, respectively, as well as a non-significant change in plasma HDL-C and NF-κB in comparison to B[a]P-treated mice.
3.4. Effect of Dihydro-cucurbitacin (DHCE) on GSH, CAT, GPx, and MDA in the Lung of Treated Mice
Lung GSH, CAT, and GPx levels were significantly decreased by 57.84, 62.36, and 78.18, respectively, as well as a significant increase in lung MDA levels by 69.41% in B[a]P-administered mice compared to the normal group (Table 6). On the other hand, when the experimental mice were treated with B[a]P and then administered DHCE (18.6 mg/kg b.w.), lung GSH, CAT, and GPx levels were significantly increased by 102.57, 74.20, and 227.08%, respectively, as well as MDA levels, which were decreased significantly by 17.26% (p< 0.05). Moreover, adminis- tration of DHCE (46.5 mg/kg.b.w.) significantly increased the levels of lung GSH, CAT, and GPx by 118.64, 155.11, and 318.75%, respectively, as well as decreased MDA levels by 34.11% compared to the B[a]P-exposed mice (p< 0.05). In addition, administration of tamoxifen non-significantly changed the levels of GSH, CAT, and MDA, as well as significantly decreased GPx levels by 18.75% compared to the B[a]P-administrated mice (p< 0.05).
No. | Groups | TC (mg/dl) |
TG (mg/dl) |
HDL-C (mg/dl) |
IL-6 (pg/ml) | NF-κB (pg/ml) |
---|---|---|---|---|---|---|
(I) | Normal control | 174.51 ± 12.45a |
113.76 ± 6.19a |
35.83 ± 2.13c |
150.62 ±14.20a |
112.80 ± 8.99 a |
(II) | B[a]P (20 mg/kg b.w.) | 236.74 ± 7.15 d |
191.17 ± 13.26e |
20.49 ± 3.05a |
209.83 ±10.98e |
369.03 ± 13.69 e |
(III) | B[a]P + DHCE (18.6 mg/kg.b.w.) |
185.13 ± 14.62b |
154.00 ± 8.67c |
25.87 ± 2.03b |
183.04 ±15.10c |
212.98 ± 14.76 c |
(IV) | B[a]P + DHCE (46.5 mg/kg.b.w.) |
178.50 ± 9.05a |
123.86 ± 6.00b |
32.27 ± 2.86c |
158.37 ±12.75b |
132.17 ±11.49 b |
(V) | B[a]P + Tamoxifen (2 mg/kg.b.w) | 200.02 ± 13.72c |
160.48 ± 7.19 d |
18.76 ± 2.29a |
251.47 ±12.72d |
397.84 ± 22.01 d |
Table 6.
No. | Groups | GSH (µmol/mg protein) |
CAT (U/mg protein) |
Glutathione peroxidase (nmol NADPH/ min/mg protein) |
MDA (nmol/mg protein) |
---|---|---|---|---|---|
(I) | Normal control | 36.89 ± 3.22 c |
15.86 ±1.63 d |
2.20 ± 0.29 e |
85.17 ±7.74a |
(II) | B[a]P (20 mg/kg b.w.) | 15.55 ± 2.96 a |
5.97 ± 0.64 a |
0.48 ± 0.07a |
144.29 ±8.66e |
(III) | B[a]P + DHCE (18.6 mg/kg.b.w.) |
31.50 ±3.37 b |
10.40 ± 1.10 b |
1.09 ±0.16c |
119.38 ±9.29c |
(IV) | B[a]P + DHCE (46.5 mg/kg.b.w.) |
34.00 ±2.41 b |
15.23 ±1.22 c |
2.01 ±0.23 d |
95.07 ±7.66b |
(V) | B[a]P + Tamoxifen (2 mg/kg.b.w) | 16.24 ± 2.91 a |
6.01 ± 0.34 a |
0.39 ±0.04b |
162.40 ± 6.75d |
3.5. Effect of DHCE on MMP-2 and MMP-12 in the Lung of Treated Mice
B[a]P-administrated mice dramatically increased their lung MMP-2 and MMP-12 by 72.36 and 74.94, respectively, compared to normal mice (Table 7). Administration of mice DHCE (18.6 mg/kg b.w.) significantly decreased the levels of MMP-2 and MMP-12 by 32.72 and 14.35 compared to B[a]P-administrated mice (p< 0.05). Moreover, giving DHCE (46.5 mg/kg.b.w.) to mice lowered their levels of MMP-2 and MMP-12 by 22.79 and 29.32 times compared to mice that had been exposed to B[a]P (p< 0.05). In addition, administration of tamoxifen significantly increased the levels of MMP-2 by 26.69%, respectively, compared to B[a]P-exposed mice (p< 0.05). Moreover, giving tamoxifen to mice that had been exposed to B[a]P did not significantly change the levels of MMP-12.
3.6. Effect of DHCE on Lung cPLA-2 and sPLA-2 Gene Expression in Treated Mice
Fig. (3) declares a significant increase of lung cPLA-2 and sPLA-2 gene expression by 902.97 and 659.40% in B[a]P-exposed mice as compared with normal mice. Administration of DHCE (18.6 mg/kg.b.w.) showed a significant decrease in the levels of cPLA-2 and sPLA-2 gene expression by 54.49 and 56.97%, respectively, as compared with B[a]P-exposed mice. Moreover, our results showed a significant decrease in cPLA-2 and sPLA-2 gene expression by 68.31 and 78.22%, respectively, in a group of mice treated with DHCE (46.5 mg/kg b.w.) as compared with B[a]P-exposed mice (p<0.05). In addition, treatment of mice with tamoxifen showed a significant increase in cPLA-2 and sPLA-2 gene expression by 31.88% and 27.38%, respectively, as compared with B[a]P-administrated mice (p<0.05).
No. | Groups | MMP-2 (ng/mg tissue) | MMP-12 (ng/mg tissue) |
---|---|---|---|
(I) | Normal control | 48.35 ±4.91a |
213.10 ±6.20 a |
(II) | B[a]P (20 mg/kg b.w.) | 83.34 ±5.07 e |
372.8 ±16.55e |
(III) | B[a]P + DHCE (18.6 mg/kg.b.w.) |
56.07 ±4.43b |
319.28 ±16.65 c |
(IV) | B[a]P + DHCE (46.5 mg/kg.b.w.) |
64.35 ±2.84 c |
263.49 ±6.36 b |
(V) | B[a]P + Tamoxifen (2 mg/kg.b.w) | 105.59 ±6.46 d |
386.14 ± 12.24 d |
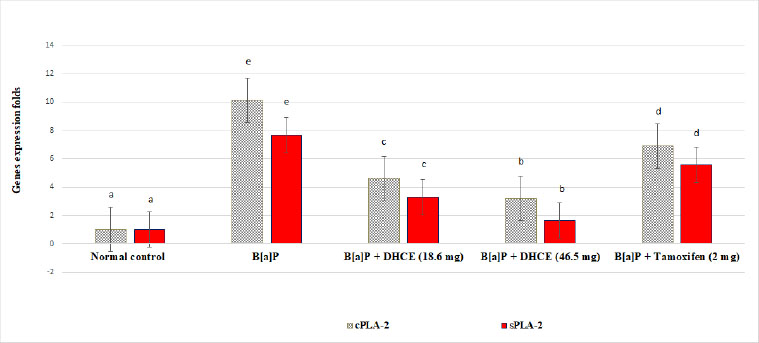
Effect of dihydro-cucurbitacin (DHCE) on lung cPLA-2 and sPLA-2 gene expression in treated mice.
3.7. Histopathological Examination
Table 8 and Fig. (4a) show a photomicrograph of lung tissues (group I). Displaying a histological feature with no histopathological alterations. Lungs with typical alveoli (A) and inter-alveolar septa (arrow). The standard epithelial cell of the bronchi is readily visible (bold arrow).
Most pulmonary airways in group (II) B[a]P-adminis- tered mice suffered significant impairment. Numerous alveoli looked deformed, with pronounced narrowing and collapse of numerous alveoli and expansion of adjacent alveoli (DA) and distended congested blood vessels (CO). Notable is the existence of a substantial mass of inflammatory cells (LI). There is a significant amount of red blood cell extravasation (EX). Most inter-alveolar septa were thickened (double-headed arrow), with areas of hemorrhage (H). Inter-alveolar septa exhibited vacuolation (wavy arrow) (Table 8 and Fig. 4b).
No. | Groups | Inflammation | Collapsed Alveoli | Widening Alveoli | Congestion | Thick Septa |
---|---|---|---|---|---|---|
(I) | Normal control | - | - | - | - | - |
(II) | B[a]P (20 mg/kg b.w.) | +++ | +++ | +++ | +++ | +++ |
(III) | B[a]P + DHCE (18.6 mg/kg.b.w.) |
++ | ++ | ++ | ++ | ++ |
(IV) | B[a]P + DHCE (46.5 mg/kg.b.w.) |
+ | + | + | + | + |
(V) | B[a]P + Tamoxifen (2 mg/kg.b.w) | ++ | ++ | ++ | + | + |
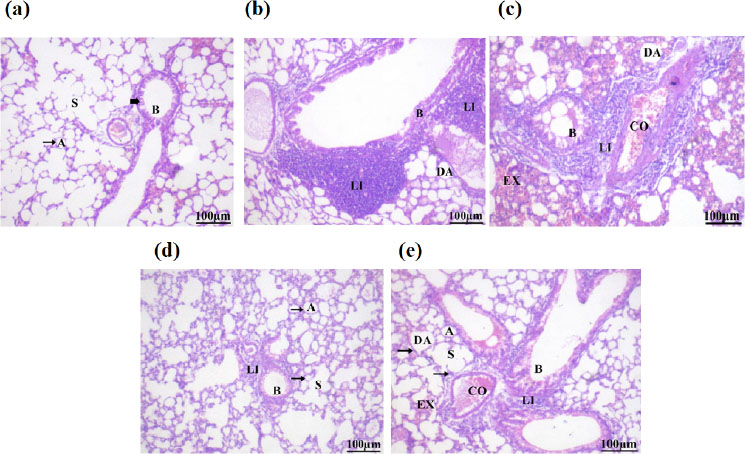
Sections stained with hematoxylin and eosin (H&E; 200 X) histological examination of mice lung tissues of different groups compared to the control group. (a): Group I: Normal control; (b): Group II: Was administrate B[a]P (20 mg/kg b.w.); (c): Group III: B[a]P + DHCE (18.6 mg/kg.b.w.); (d): Group IV: B[a]P + DHCE (46.5 mg/kg.b.w.); (e): Group V: B[a]P + Tamoxifen (2 mg/kg.b.w).
Abbreviations: Alveolar lumina (A), pneumocyte-lined alveolar sacs (S), simple columnar epithelium-lined bronchioles (B), inter-alveolar septa (arrow), standard epithelial cell of the bronchi (bold arrow), a substantial mass of inflammatory cells (LI), expansion of adjacent alveoli (DA), distended congested blood vessels (CO), red blood cell extravasation (EX)
In group (III), B[a]P-exposed mice treated with DHCE (18.6 mg/kg b.w.), relative to the positive group, there was minor protection of the lungs. Significant inter-alveolar infiltration (LI) and many alveoli dilations (DA). The interalveolar septa appeared to have thickened, as indicated by the double-headed arrow. We found significant RBC extravasation (EX) in the alveolar cavities (Table 8 and Fig. 4c).
In group IV, a, B[a]P-exposed mice treated with DHCE (46.5 mg/kg b.w.), most regions of the lung sections displayed normal architecture with normally appearing alveoli (A) with thin inter-alveolar septa (arrows) and a moderate level of cellular infiltrations, indicating remarkable preservation as compared with the positive group. The bronchi exhibited their usual interior epit- helium and bronchiolar anatomy. While the majority of interalveolar septa are thin (arrows), we still observed thickened septa (double-headed arrow). The configuration of alveolar sacs (S) and alveoli (A) is normal. Healthy simple columnar epithelium surrounds the bronchiole (B). Minorly congested blood vessels were observed (Table 8 and Fig. 4d).
In group (V), B[a]P-exposed mice treated with tamoxifen (2 mg/kg b.w.) demonstrated relative improve- ment in lung architecture but not full histological repair. It was seen that the bronchioles were dilated (B), there was moderate inflammatory cell infiltration in the moderately thickened septa (double-headed arrow) surrounding the alveoli and bronchioles, there were limited areas of smaller alveoli with enough dilatation (DA) of nearby alveoli, and some alveoli that looked normal (A) (Table 8 and Fig. 4e).
4. DISCUSSION
Biotransformation of cucurbitacin E glucosides by C. lunata NRRL 2178 and evaluation of their anticancer activity as well as estimation of the lung protective activity of the DHCE against benzo[a]pyrene-induced lung toxicity in mice were investigated in the present study.
Phase I clinical trials are currently investigating the antitumor effectiveness of several triterpenoids [41]. In addition, several reviews proved the microbial modification of terpenoids [42-44].
In the present study, Curvularia lunata efficiently metabolized curcrbitacin-E-2-D-glucoside into two main metabolites: cucurbitacin-E and DHCE. We used solvent extraction to isolate the metabolites, followed by purification using column chromatography. We evaluated the metabolite yields using 1H-NMR and C13-NMR spectroscopy.
The 1H-NMR and C13-NMR spectra showed that the glucose part of curcrbitacin-E-2-D-glucoside was taken away, and the structure of the aglycone did not change in any other way. 1H-NMR spectral data of cucurbitacin-E proved that no glucose moiety signals appeared in the area between δ 5.43 and 5.76 and the presence of two trans-olefinic protons (H 23 and 24) at δ 7.3–7.4. The three-proton signal at 1.22 ppm confirmed the presence of the 25-acetate functional group, and a new signal at 8.2 ppm was assigned to the carbon methine proton at C-24.
In addition, 1H-NMR data was supported by C13-NMR, which proved the absence of signals for the glucose moiety between 60.28 and 102.33 ppm.
1H-NMR of DHCE indicated that it lacked the signals of the glucose moiety as well as olefinic protons H-23 and -24. Moreover, the three carbonyl signals at 120.9, 125.6, and 173.45 ppm were assigned to C-3, C-11, and C-22, respectively. The C-22 signal's downfield shift revealed the saturation of the C23-C24 double bond [45].
The absence of the glucose moiety in all metabolites suggests that glycolysis was the first step in the biotrans- formation sequence, followed by secondary biotrans- formation (Fig. 1). Curvularia lunata is a well-known biocatalyst that hydroxylates a variety of steroids at various locations. However, the remarkable biotrans- formation only occurred on the side chain, not on the main cyclic structure of cucurbitacin [46]. Fig. (1) illustrates the possible biotransformation sequence based on the identification of these metabolites.
Following glucose cleavage with CE, reduction of the conjugation 23–24 doubly bound would produce a saturated side chain to yield DHCE.
The present study investigated the anticancer activity of DHCE against A549. Researchers investigated the anticancer potential of DHCE against different cells [47, 48].
DHCE might increase cells in the G2/M phase of the cell cycle, delaying mitosis. We achieved cell cycle arrest by downregulating the production of CDC2 and cyclin B1 proteins, which in turn dissociated the CDC2/cyclin B1 complex via GADD45 upregulation [49]. Moreover, it might suppress the levels of cyclin A2, cyclin B1, and cyclin E1, resulting in an accumulation of cells in the G1/G0 phase of the cell cycle [50].
The results of the current investigation revealed that the LD50 of DHCE in mice was 930 mg/kg b.w. The main toxicological effect of DHCE in experimental mice appears to be an increase in capillary permeability, irritation of the intestinal mucosa, and a strongly increased intestinal motility. The mice die from congestion of the intestine, pancreas, liver, and kidneys. We used 1/50 and 1/20 LD50 of DHCE to evaluate its lung protective activity against B[a]P-induced lung cancer in mice.
According to reports, B[a]P may be a hydrocarbon that contributes to the development of lung cancer [51].
Moreover, monitoring tumor progression to identify therapy options and responses is a common mechanism for the management of lung cancer [52].
However, chemotherapy is still the only treatment option for lung cancer. Moreover, chemotherapeutics have side effects like hemopoietic tissue damage and immuno- logical suppression, which can make it hard to take medicine or cause the patient to stop therapy [53].
Currently, cytokines and free radical suppression, as well as antioxidant induction, are widely suggested as key strategies for protecting cells from immune suppression and external carcinogens [54]. Researchers increasingly acknowledge the beneficial effects of phytochemicals with antioxidative capabilities in cancer prevention and management, commonly known as chemoprevention [55]. Chemoprevention is now the most promising approach for significantly decreasing the occurrence of lung cancer, especially in cases of B[a]P-induced lung cancer [34].
Elevation of plasma TC and TG, as well as depletion of HDL-C, occur because of several abnormalities in physiological events during carcinogenesis. This could be caused by anorexia and malabsorption. It is an indication of chemically induced organ oxidative stress and toxicity [53-55]. This study significantly reduced HDL-C in B[a]P-stimulated mice. Furthermore, B[a]P-activated animals had higher plasma TC and TG levels, which could be attributed to lung abnormalities [54].
Prophylactic treatment with DHCE at both doses, particularly 46.5 mg/kg body weight, restored the lipid profile levels in mice. We found the therapeutic activity of DHCE on plasma TC, TG, and HDL-C to be near normal, suggesting DHCE's protective benefits against lung cancer. The effect of DHCE is more pronounced than that of tamoxifen.
B[a]P is a carcinogen that generates many free radicals. Extreme radicals react with lipids to release MDA. MDA is a reactive chemical that can interact with DNA to cause mutations and cancer [54]. Currently, B[a]P-activated mice showed a significant increase in MDA compared to the control group. The prophylactic effect of DHCE treatment in B[a]P-triggered mice diminished the status of MDA considerably. Enzymatic antioxidants (GPx and CAT) and non-enzymatic GSH can elucidate this by eradicating MDA.
Using both short-term and long-term models of inflammation, Khayyal et al. studied how Iberis amara extracts (which are high in cucurbitacins) could reduce inflammation and protect cells from damage [56]. Because cucurbitacins B, E, and I are in the extract, both models showed a decrease in inflammation that was related to the dose. This supports the idea that the extract has strong anti-inflammatory properties [57]. Our results were in line with several articles that proved the antioxidant activity of certain medicinal plants due to the presence of cucurbitacins [58, 59].
Cancer development depends in large part on chronic inflammation. Two key proteins, IL-6 and NF-B, regulate inflammation-related cancers. While IL-6 is a crucial component of cell development and cell variety, NF-κB is the cause of tissue inflammation.
Similarly, MMP-2 and MMP-12 play a vital role in inflammation and are required for acute cigarette smoke-induced connective tissue breakdown [60]. A number of studies [61, 62] have also shown that cultured macrophages from the lavage fluid of human smokers or from animals exposed to smoke have higher electrolytic activity. Human lungs with emphysema also exhibit higher levels of MMP-2 and MMP-12 than lungs without the disease [63].
The lungs of B[a]P-treated mice contained significantly more proinflammatory mediators, such as IL-6, NF-B, MMP-2, and MMP-12, compared to the control group. In B[a]P-induced animals, Preventive DHCE treatment returned this state to normal. This was unmistakably confirmed by histological findings that showed reduced harm in the lung alveolar, pointing to the antitumor effect of DHCE against experimental lung cancer caused by B[a]P.
DHCE's anti-inflammatory and antiproliferative effects were also caused by its role in the polymerization of the actin cytoskeleton [64]. We demonstrated that DHCE not only reduced B[a]P-induced inflammation, but also lowered the levels of IL-6, MMP-2, and MMP-12. A lower level of NF-κB protein showed that DHCE also slowed down lung apoptosis in rats that had been given B[a]P. Furthermore, studies have reported that DHCE exhibits protective properties in a model of various diseases by regulating cytokine pathways [65]. Consequently, our study revealed that DHCE improved lung injury and attenuated inflammation impairment, including cytokine inhibition and increased antioxidant biomarkers. These results showed that DHCE improved B[a]P-induced lung cancer in mice.
In the present investigation, we observed a striking increase in inflammatory mediators as well as a decrease in the level of antioxidant biomarkers in mice treated with B[a]P. The statistical analysis and histopathological examination clearly showed that this reflects systemic inflammation.
In the current study, B[a]P administration over- expressed cPLA2, stimulating the lung cells to produce and biosynthesize arachidonic, lipoxygenases and cyclo- oxygenases then transferred to eicosanoids [66], including prostaglandins [67]. Eicosanoids such as the cytokines IL-6 and NF-κB serve as chemoattractants for neutrophils, playing a key role in the inflammatory process [68] (Chart 1). In addition, the broken cell membrane moved the lung cell's released IL-6 and NF-B into the cytoplasm, which led to an increase in sPLA2 levels [69]. Moreover, overexpression of sPLA2 led to surfactant catabolism, oxygenation impairment, and acute respiratory distress syndrome (ARDS) [24]. Surprisingly, we discovered that DHCE lowers the expression of cPLA2 and sPLA2 in B[a]P-induced cells. This lowers the cytokine storm, which is the main cause of ARDS and lung cancer.
As a result, cancer therapy that uses apoptosis induction and cycle arrest of cancer cells is effective [70]. In this study, DHCE treatment of lung toxicity cells resulted in improvement of oxidative stress biomarkers and inflammatory mediators, typical signs of cell recovery [71]. In addition, DHCE's anti-inflammatory and anti- oxidant effects depend on the dose. It does this by decreasing the production of proapoptotic proteins and restoring normal levels of enzymatic and non-enzymatic antioxidant biomarkers.
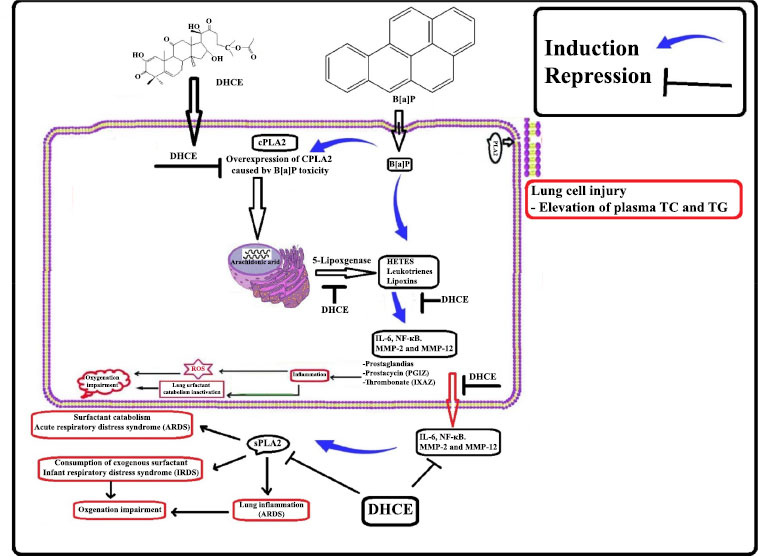
The perspective mechanism of action for DHCE.
Our results established the cytotoxic potential of DHEC in humans against the lung cancer cell line A549. Consistently, the administration of DHEC led to a decrease in ROS and cytokine production, as well as a downregulation of cPLA2 and sPLA2. The combined information from in vitro and in vivo research has successfully identified the advantages of DHEC in the treatment of lung cancer. An increase in antioxidant enzymes indicates the activation of intrinsic apoptosis, thereby reinforcing the cytotoxicity evidence.
The current study's histological results demonstrated that DHCE influenced the protection of the lung structure in mice suffering from lung cancer due to exposure to B[a]P. Treatment with DHCE significantly reduced the severity of toxicity and stellate infiltration compared with the B[a]P-treated control group.
Our biochemical, molecular biological, and histological results also showed that DHCE may help protect the lungs of mice from lung toxicity caused by B[a]P.
To our knowledge, DHCE, a cucurbitacin-E-glucoside metabolite of C. lunata NRRL 2178 biotransformation, has never been documented as a protector against B[a]P-induced lung damage, and this investigation may be the first of its kind.
CONCLUSION
Overall, the current study on DHCE showed well measured in vitro and in vivo investigations that suggest beneficial effects on lung cancer brought on by B[a]P and inflammatory oxidative damage. Therefore, these preli- minary findings suggest DHCE as a potential lung cancer chemoprevention candidate. Future studies will be necessary to clarify the potential molecular processes essential to the apparent anticancer action.
AUTHORS’ CONTRIBUTION
Experimental design for the NRRL 2178 biotrans- formation of cucurbitacin-E-glucoside was carried out by Prof. Mahmoud Abdel-Mongy and Dr. Fotna Magdy. Prof. Tamer Roshdy and Prof. Ahmed Salah conducted in vitro and in vivo studies of the obtained metabolite, DHCE. The biochemical study was carried out by Prof. Hussein MA. We wrote the first draft of the manuscript, managed the analyses of the study, and managed the literature searches carried out in collaboration with all authors.
LIST OF ABBREVIATIONS
DHCE | = dihydro-cucurbitacin-E |
TC | = Total Cholesterol |
GSH | = Glutathione |
MMP-2 | = Matrix Metalloproteinases-2 |
sPLA2 | = Secretory Phospholipase A2 |
IL-6 | = Interleukin-6 |
GPx | = Glutathione Peroxidase |
ETHICS APPROVAL AND CONSENT TO PARTICIPATE
The experiment received approval from the Ethics Committee of the Faculty of Applied Health Science and Technology, Egypt (Reference number 20221025).
HUMAN AND ANIMAL RIGHTS
The animal research techniques were conducted according to the guidelines specified in the ninth edition of the Guide for the Care and Use of Laboratory Animals issued by the National Academy of Sciences, National Academies Press, Washington, D.C./ This study adheres to internationally accepted standards for animal research, following the 3Rs principle. The ARRIVE guidelines were employed for reporting experiments involving live animals, promoting ethical research practices.