All published articles of this journal are available on ScienceDirect.
Genetic Variants of Multidrug-Resistant Klebsiella pneumoniae Isolated from Al-Ramadi Teaching Hospital, Iraq
Abstract
Background
K. pneumoniae stands as a prominent contributor to hospital outbreaks on a global scale. Furthermore, there is a rising trend of antibiotic-resistant strains of K. pneumoniae causing severe and potentially fatal invasive infections, leading to increased morbidity and mortality. Thus, knowledge of the genetic relatedness of this bacterium is necessary to solve this problem using the BOX–PCR technique.
Objective
This research aimed to determine the genetic relatedness of nosocomial K. pneumoniae isolated from different clinical sources with antibiotic resistance.
Materials and Methods
Between March 2021 and June 2021, a collective of 45 nosocomial K. pneumoniae isolates were obtained from various sources. Additionally, all isolates underwent diagnosis through both conventional tests and an automated system, specifically the VITEK-2. In the present study, six antibiotics from different classes were examined for activity against K. pneumoniae using Kirby–Bauer disk diffusion. DNA was extracted from fifteen isolated using Wizard™ Genomic DNA Purification Kit. Thus, BOX-PCR was used to identify the genetic relatedness of this bacterium.
Results
According to the source of samples, they were distributed to 25/45 (55.56%) sputum, 15/45 (33.33%) urine, 3/45(6.67%) wound, and 2/45(4.44%) blood. The antibiotic susceptibility profile revealed high resistance rates, notably against 95.5% Piperacillin-tazobactam, 80% Ceftriaxone and Ceftazidime, and 75.6% Cefepime. Of the 45 K. pneumoniae isolates analyzed, a significant proportion exhibited resistance to multiple antibiotics, with 33.33% classified as MDR strains. Molecular typing using BOX repetitive sequences demonstrated a high degree of genetic variability among the isolates, with the presence of unique genotypes and strong clonality observed. Dendrogram analysis showed the genetic relationship among antibiotics-resistant K. pneumoniae isolates.
Conclusion
The current work showed that the BOX-PCR technique is necessary for the investigation of the genetic diversity of K. pneumoniae, and it is an easy, reproducible, fast, and cost-effective tool.
1. INTRODUCTION
It is a gram-negative bacterium with a capsule, is responsible for approximately 10% of nosocomial infections. It causes various infections, including pneumonia, infected burns, urinary tract infections (UTIs), septicemia, and meningitis [1]. Infections caused by antibiotic-resistant K. pneumoniae are challenging to treat due to its resistance to a wide range of drugs, including β-lactams. Addressing infections caused by antibiotic-resistant K. pneumoniae poses a significant challenge due to the bacterium's inherent and acquired resistance to a broad spectrum of drugs, such as β-lactams. Managing MDR strains can prove particularly daunting, particularly for elderly individuals, those with compromised immune systems, or infants with immature immunity [2].
While K. pneumoniae is traditionally known to be acquired in hospital settings and primarily affects immunocompromised individuals as an opportunistic pathogen, hypervirulent and multidrug-resistant (MDR) strains have arisen due to the presence of an extensive accessory genome comprising both plasmids and chromosomally-encoded genes [3]. Globally, hospital-acquired infections have been documented at a prevalence of 8.7%, with approximately 10% attributed to K. pneumoniae. This bacterium harbors genes responsible for multidrug resistance, which can be transferred to other gram-negative bacteria. Multidrug-resistant (MDR) K. pneumoniae strains are implicated in various infections, such as lower respiratory tract infections, bacteremia, bloodstream infections, wound infections, and urinary tract infections [4]. The challenges linked to MDR K. pneumoniae infections encompass treatment strategy shortcomings, prolonged hospitalization, heightened medical expenses, and elevated rates of mortality and morbidity [5].
The widespread use of antimicrobial agents in healthcare facilities has led to the emergence of resistant bacterial pathogens, leading to increased rates of illness and death worldwide. Klebsiella, known for its resistance, is one of the opportunistic pathogens that commonly acquire antibiotic resistance, accounting for around one-third of all Gram-negative infections [6]. These encompass ailments, such as bloodstream infections, pneumonia, urinary tract infections (UTIs), and infections acquired both in hospitals and communities. Current studies suggest that Klebsiella has acquired resistance to most frequently prescribed antibiotics, including cephalos- porins, monobactams, fluoroquinolones, and aminoglyco- sides. This resistance is linked to the frequent empirical administration of antibiotics and the ongoing exposure of Klebsiella to diverse antimicrobial agents, facilitating the emergence of resistant strains [7]. Currently, the surge in infections caused by Multi-Drug Resistant (MDR) K. pneumoniae presents a formidable obstacle owing to restricted antibiotic choices. This results in increased morbidity, extended hospitalization periods, higher mortality rates, and augmented healthcare costs in comparison to infections induced by antibiotic-sensitive microorganisms.
Nevertheless, the prevalence of antibiotic-resistant bacteria fluctuates significantly depending on geogra- phical location, national policies, and vulnerable demographics. The gravity of this challenge is intricately tied to the efficacy of measures deployed to mitigate the dissemination of drug-resistant bacteria [8]. The existence of resistance within pathogens carries considerable implications, impacting both clinical results and economic burdens. These consequences encompass prolonged hospitalizations, increased expenditures associated with hospital care and antimicrobial therapies, and heightened mortality rates. Recognizing the importance of monitoring pathogens as they develop resistance to antibiotics becomes apparent, especially when considering the risk of severe sepsis. Such surveillance plays a critical role in shaping antibiotic strategies to guarantee the accessibility of optimal treatment choices [9].
In contemporary healthcare environments, K. pneumoniae is recognized as the second most commonly encountered Gram-negative pathogen, following Escherichia coli. The prevalence of multidrug-resistant organisms (MDR) or superbugs has escalated, leading to heightened concerns and more severe consequences [10]. Two molecular typing methods are described as common methods in molecular typing for K. pneumoniae, including multi-locus sequencing typing and pulsed-field gel electrophoresis [11]. Rep-PCR is a quick, simple, reliable genotyping method and an inexpensive technique [12]. It is used to differentiate between bacteria that are closely related strains [13]. It can be classified into BOX elements and (ERIC) sequences. The Enterobacterial Repetitive Intergenic Consensus Polymerase Chain Reaction (ERIC-PCR) is a molecular method utilized to evaluate genetic diversity within the Enterobacteriaceae family. ERIC sequences, typically 126 base pairs in length, are noncoding and exist as conserved regions. This technique effectively identifies genetic variations among bacterial isolates, offering a rapid, precise, and dependable means of assessing their genetic similarity [14]. Moreover, BOX PCR is considered a mosaic repetitive element made up of three other subunit sequence combinations, including boxA (59 nucleotides), boxB (45 nucleotides), and boxC (50 nucleotides) [15]. This current research aimed to determine the genetic relatedness of nosocomial K. pneumoniae isolated from different clinical sources with antibiotic resistance.
2. METHODS
2.1. Bacterial Strains
From March 2021 to June 2021, a total of 45 nosocomial K. pneumoniaewere isolated from different sources, such as urine, sputum, and wound. Isolates were gathered from a range of hospitals across Anbar, such as Al-Ramadi Teaching Hospital, Al-Ramadi Teaching Hospital for Maternity and Pediatrics Hospital, the Onco- logy Center, Al-Safwa Private Hospital, Al-Razi Private Hospital in Al-Ramadi city, Al-Rashid Hospital in Khalidiya city, Al-Fallujah Teaching Hospital in Fallujah city, Hit Hospital, and Hadeetha Hospital.
2.2. Inclusion and Exclusion Criteria
Specimens were gathered from men residing in Anbar province, afflicted with bacterial inflammation, aged 35 and above, married, and without chronic ailments like diabetes or coronary artery disease, among others.
2.3. Cultivation and Identification of Klebsiella pneumoniae
Additionally, all isolates underwent identification using traditional methods, such as culture media (on macConky agar, blood agar (HiMedia; India), gram stain microscopy, and biochemical assays [16, 17], alongside an automated approach employing the VITEK-2 compact system. The final identification of the bacterial isolate was achieved using the VITEK-2 (GN cassette) system, which employs established biochemical tests for Gram-negative identi- fication and features a comprehensive database for routine tests. A bacterial suspension with a density of 0.5 to 0.63 McFarland was prepared in 3 ml of normal saline using DensiCHEK. Analysis was conducted utilizing the VITEK®2 software system 07.01, adhering to the manufacturer's guidelines (bioMèrieux, Marcy l’Etoile, France). Subsequently, the isolated strains were preserved at -20°C in brain heart infusion broth supplemented with 20% glycerol for further analysis.
2.4. Susceptibility Test
Kirby–Bauer method [18, 19] and automated sensitivity by vitek-2 compact technique were used to know antibiotic resistant-bacteria.
Kirby-Bauer method was used for antibiotic susceptibility tests for six different antibiotics, including Imipenem, Cefepem, Ceftriaxone, Amikacin, Ciprofloxacin, and Piperacillin-tazobactam (Merck; Germany). Based on Clinical Laboratory Standard Institute (2020) and EUCAST [20], the following technique (antibiotic susceptibility test) was used: Two colonies were incubated in nutrient broth at 37°C overnight to achieve 0.5 McFarland turbidity (1.5×10^8 CFU/ml). The bacterial suspension was streaked on Muller Hinton agar (HiMedia; India) , and antibiotic disks were placed on the surface. Plates were incubated at 37°C for 24 hours, and inhibition zone diameters were measured according to CLSI 2020 guidelines [21].
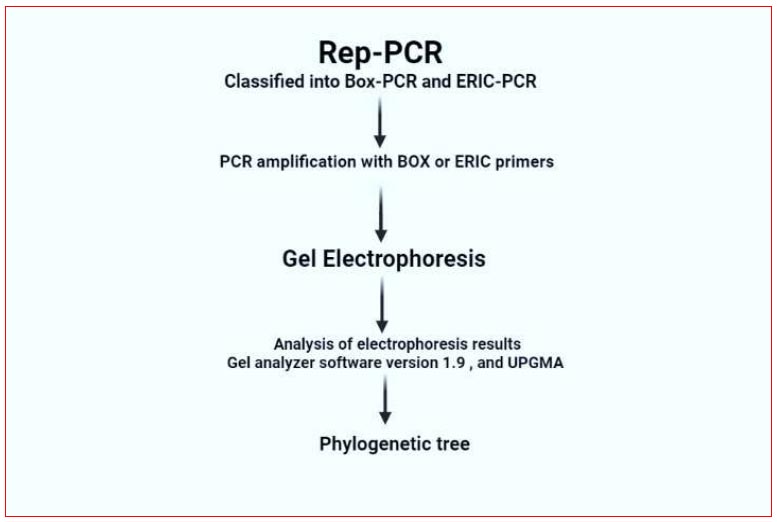
Processing steps of Rep-PCR technique.
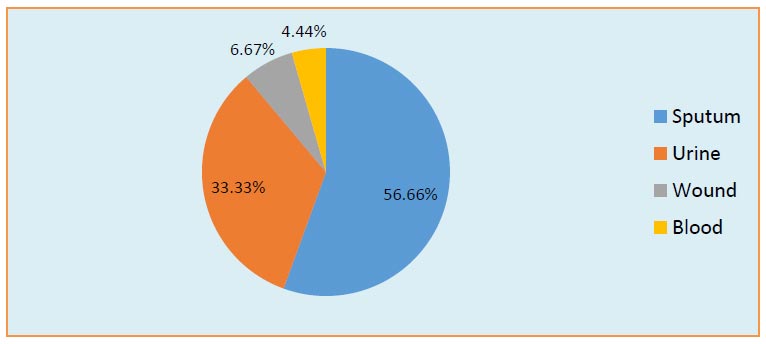
Distribution of clinical K. pneumoniae isolates.
2.5. Extraction of Bacterial DNA
K. pneumoniae isolates were incubated in brain heart infusion broth (HiMedia; India) for 18 hours at 37°C. Bacterial DNA was extracted from 15 K. pneumoniae isolates by Wizard™ Genomic DNA Purification Kit (Promega Corporation, USA) according to the manufacturer’s instructions. Fifteen bacterial isolates were selected for various reasons, including their multidrug resistance and production of Extended-spectrum β-lactamase enzymes.
2.7. BOX-PCR Protocol
2.7.1. Primer Sequence and Dilution
BOX-PCR fingerprinting used the primer BOXA1R (5'-CTACGGCAAGGCGACGCTGACG-3') from Macrogen (Korea), resuspended in nuclease-free water to a final concentration of 10 picomols/µl [22].
2.8. Reaction of PCR
The 20 μl reaction mixture containing 5 μl of master mix, 3 μl of primer, 3 μl of template DNA, and 9 μl of water from Promega Corporation, USA, was employed.
2.9. PCR Program
The procedure involved an initial pre-denaturation step at 94°C for 5 minutes, followed by 35 cycles consisting of denaturation at 94°C for 1 minute, primer-annealing at 40°C for 2 minutes, extension at 72°C for 2 minutes, and a final extension at 72°C for 10 minutes.
2.10. Agarose Gel Electrophoresis
The PCR products underwent electrophoresis on a 1.5% agarose gel in 1x TAE buffer. Ethidium bromide (0.5 mg/mL) was used to stain the gel, which was then illuminated with UV light. Additionally, each gel included a 1 kb DNA ladder sourced from Thermo Fisher Scientific Company, USA.
2.11. Gel Electrophoresis Products (Cluster) Analysis
The banding patterns generated by BOX-PCR were analyzed utilizing Gel Analyzer software, version 1.9. Dendrograms were constructed using the UPGMA method [23].
2.12. Discriminatory Index
The discriminatory index, Simpson’s index of diversity (D), was computed using the following formula:

In this formula, N represents the total number of isolates in the sample population, s denotes the total number of described types, and nj indicates the number of strains belonging to the jth type. The Simpson's index of diversity varies between 0 and 1, where a value of 1 indicates high discrimination, while a value of 0 suggests a lack of discrimination.
3. RESULTS AND DISCUSSION
3.1. Isolation and Identification
All samples were collected over two months, from the beginning of March to the end of June 2021, and were from patients of both genders. The 45 clinical samples were isolated from different sources, including urinary tract infections, wounds, sputum, and blood. As shown in Fig. (2), in the current study, samples were distributed according to the source of infection to 25/45 (55.56%) sputum, 15/45 (33.33%) urine, 3/45(6.67%) wound, and 2/45(4.44%) blood. Numerous studies agreed with our study that K. pneumoniae is responsible for 6-17% of UTIs [24-26], 7-14% of pneumonia [24, 25], 4-15% of septicemia [27-30], 2-4% of wound infections [25, 26, 31], 4-17 nosocomial infections in intensive care units [25, 26, 32], and 3-20% of all neonatal septicemia cases [33-35].
Particularly noteworthy among Klebsiella species with medical relevance is K. pneumoniae, which significantly contributes to hospital-acquired infections, such as urinary tract infections, pneumonia, septicemias, and soft tissue infections. The primary sources facilitating the transmis-sion of Klebsiella are the gastrointestinal tract and the hands of healthcare personnel. Due to their rapid spread within the hospital environment, these bacteria frequently instigate nosocomial outbreaks. Outbreaks of multidrug-resistant Klebsiella spp., particularly in neonatal wards, often stem from novel strains known as extended-spec-trum-β-lactamase (ESBLs) producers [36]. Over the recent years, there has been a consistent rise in the occurrence of extended-spectrum-β-lactamase (ESBL)-producing strains within clinical Klebsiella isolates. The resulting constraints on available therapeutic options necessitate the development of novel strategies for managing Klebsiella infections in hospital settings. While various detection methods, including conventional PCR, prove valuable for epidemiological purposes, recent insights into Klebsiella virulence factors and ESBL production offer new perspectives on the pathogenic mechanisms of these bacteria. Klebsiella pathogenicity factors, such as capsules or lipopolysaccharides, biofilm formation, and ESBL production, are currently being recognized as promising candidates for vaccination initiatives, serving as immunological measures for infection control [37].
All isolates were diagnosed using morphological examination, microscopic examination, biochemical test, and vitek -2 system, as shown in Table 1.
3.2. Susceptibility Test
Forty-five K. pneumoniae isolates from wounds, burns, sputum, and urinary tract infections were tested for antibiotic sensitivity using the Kirby-Bauer disk diffusion method [21]. The susceptibility was tested on 6 antimicrobials, including Imipenem, Cefepem, Ceftriaxone, Amikacin, Ciprofloxacin, and Piperacillin-tazobactam. The resistance percentages of 45 isolates were as follows: Piperacillin– tazobactam 95.5%, Ceftriaxone 84.44%, Cefta-zidime 80%, Cefepeme75.6%, Imipenem 64.44%, Amikacin 60%, and Ciprofloxacin 60%, as shown in Fig. (4).
In the present study, about 15/45 (33.33%) of the K. pneumoniae clinical isolates were found as MDR- K. pneumoniae, which is a very alarming health situation prevailing in Iraq. The global rise in MDR and XDR K. pneumoniae is influenced by several factors: (i) dissemination of high-risk global multiresistant genetic strains, (ii) acquisition of effective multiresistant plasmids, and (iii) acquisition of resistance genes on successful transposons [38, 39].
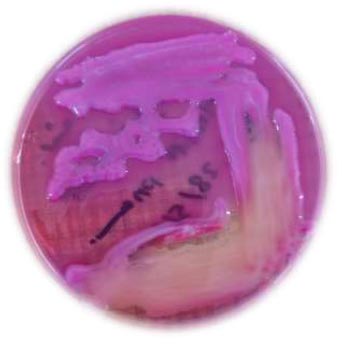
Phenotypic identification of K. pneumoniae on MacConkey agar medium.
Characteristics | K. pneumoniae |
---|---|
Blood agar | γ hemolytic, grey-white, mucoid colonies |
Catalase | +ve |
Citrate | +ve |
Gram stain | -ve |
Indole | -ve |
MacConkey agar medium | Pink colour, lactose fermenter, and mucoid colonies, as shown in Fig. (3). |
Methyl Red | -ve |
Oxidase | -ve |
Shape | Rod |
Urease | +ve |
VP | +ve |
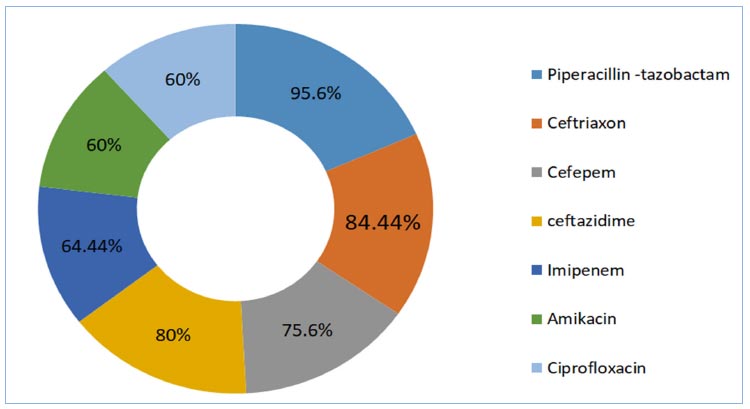
Antibiotics resistance percentage antibiogram for K. pneumoniae isolates according to Kirby-Bauer disk diffusion.
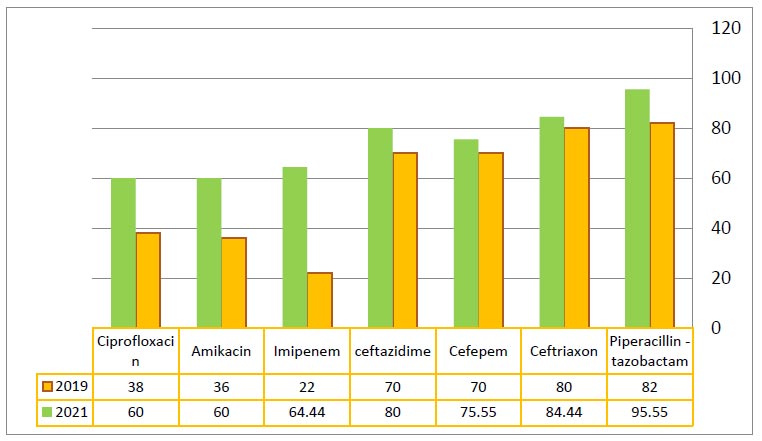
Development of antibiotic resistance against K. pneumoniae from 2019 to 2021.
Many previous studies in Ramadi city reported increasing antibiotic resistance of K. pneumoniae from 2019 to 2021 [1, 40-44], as shown in Fig. (5). The results revealed variations in the resistance of isolates to all antibiotics used in the current study. The increasing bacterial resistance to several antibiotics is considered a tremendous therapeutic problem. One of the most important factors contributing to antimicrobial resistant is the misuse and overuse of antimicrobials. Other factors include a lack of access to safe drinking water, sanitation and hygiene (WASH) for both animal and human, control in health-care facilities and ineffective infection- and disease-prevention and farms, a lack of access to high-quality, vaccines, affordable medicines, and diagnostics, and a lack of enforcement of antimicrobial resistance legislation. In the present study, the profile of antibiotic resistance among K. pneumoniae isolates was highly variable, and 83.33% of the isolates were multidrug resistant (MDR) that agreed with previous studies [14, 45-47].
Several studies have explored β-lactam resistance in Al-Anbar province. Mohammed and Al-Meani (2019) revealed elevated resistance rates, with Ceftriaxone (80%), Ceftazidime (70%), Cefepem (70%), Ciprofloxacin (38%), Amikacin (36%), and Imipenem/Meropenem (22%) demonstrating particularly high levels of resistance [1]. Yusir and Ali reported findings indicating resistance in E. coli, with notable percentages observed for Ceftriaxone (86%), Cefepime (70%), and Ciprofloxacin (62%) [42]. Another study conducted by Shiamaa and Myada reported K. pneumonia (25/75) from 75 clinical strains that were resistant to the cephalosporins community of β-lactam. They were resistant to most antibiotics under test, and they showed an elevated resistance to various groups of β-lactam and non-β-lactam antibiotics [43]. In vitro results indicate that a broad spectrum of beta-lactams, aminoglycosides, quinolones, and other antibiotics exhibit effectiveness in treating Klebsiella infections [48-50]. The clinical K. pneumoniae isolates underwent anti- microbial sensitivity testing, revealing a predominant multidrug-resistant profile. In instances of such resistance, there is an increased risk of the disease advancing to a state of permanent debilitation or even mortality for the patient if prompt isolation, identification of the causative agent, and subsequent antimicrobial susceptibility testing are not conducted during the early stages of the disease.
Furthermore, the present findings align with the outcomes of Mustafa's study in 2018, which indicated that K. pneumoniae resistance, sourced from diverse clinical cases and multiple hospitals in Baghdad, stood at 32% and 84% against ceftazidime and cefotaxime, respectively. This correlation is also consistent with the research conducted by Zedan-Alobadi, who investigated K. pneumoniae resistance in various hospitals in Baghdad. The study revealed resistance rates of 2.5% for Imipenem, 95% for amox-clav, 97% for amoxicillin, and 77.5% for ceftriaxone [51].
These findings are consistent with a prior investigation conducted by Akpaka and Swanston, where the clinical results for Escherichia coli isolates resistant to ampicillin showed a rate of 93.3% [52]. The K. pneumoniae isolates exhibited the highest antibiotic resistance levels within the cephalosporin category, ranging from 58.3% to 83.3%. In contrast, a separate study conducted in Saudi Arabia revealed that K. pneumoniae displayed the utmost resistance against ampicillin, reaching 100% [53]. The elevated resistance observed against cephalosporins, including cefazolin, ceftazidime, and cefepime, is likely attributed to the widespread prevalence of ESBL-producing Enterobacteriaceae isolates in hospitals within Erbil city. This aligns with the findings of a prior study by Haji and colleagues, who reported that 76.2% and 78.9% of Escherichia coli and K. pneumoniae isolates, respectively, were identified as ESBL-producers [53]. In 2019, Ahmed noted a swift and escalating emergence of resistance against carbapenems. The antibiotic sensitivity profile revealed resistance rates of 13.5% for imipenem and 14.86% for meropenem in Escherichia coli isolates. Concurrently, Klebsiella pneumoniae isolates demons- trated a resistance rate of 33.3% against both imipenem and meropenem [54]. Several studies have consistently demonstrated elevated levels of resistance, primarily in β-lactam antibiotics. In a study conducted by Mohammed Obaid and Safaa Al-Meani in 2019, K. pneumoniae exhibited high resistance rates, specifically 78% to Ceftriaxone, 68% to Cefpodoxime, 62% to Ceftazidime, 62% to Cefepime, and 16% to Cefoxitin [40]. In a systematic review and meta-analysis conducted by Heidary et al. in 2018, findings indicated a relatively elevated prevalence of drug-resistant K. pneumoniae isolates in Iran [55]. In their review, the highest resistance rates among K. pneumoniae isolates were to ampicillin (82.2%), aztreonam (55.4%), and nitrofurantoin (54.5%). This study found resistance rates of 52% to SXT, 51% to CTX, and 43% to both FEP and CRO. Khamesipour et al. (2016) reported widespread resistance to CRO (41.1%), SXT (36.7%), AN (32.2%), FEP (34.4%), and GM (26.7%) [56] . In the study by Moghadas et al. (2018), antibiotic resistance rates were: IPM (7.5%), CIP (16.1%), SXT (32.9%), FEP (34.1%), AN (36.4%), and CAZ (42.7%) [57]. Unlike our study, which found 67% of K. pneumoniae isolates to be MDR, Hou et al. (2015) reported 89.5% [8].
3.3. Molecular Typing of K. pneumoniae
Based on the research team's knowledge, this work was reported to the Anbar government for the first time and explained the relationship between genetic fingerprinting and antibiotic resistance. Infections caused by K. pneumoniae include UTI, blood, wound, and respiratory infections [58]. The nosocomial infections study needs genotyping. BOXA1R is a good bacterial differentiation technique. Genetic fingerprinting of MDR K. pneumoniae isolates using BOXA-1R-PCR generated 4–25 bands ranging from 216 to 1971 bp, illustrated in Fig. (6). The results showed that there are many bands in all bacterial isolates, where these isolates differed in the containment of the bands in terms of the presence of some
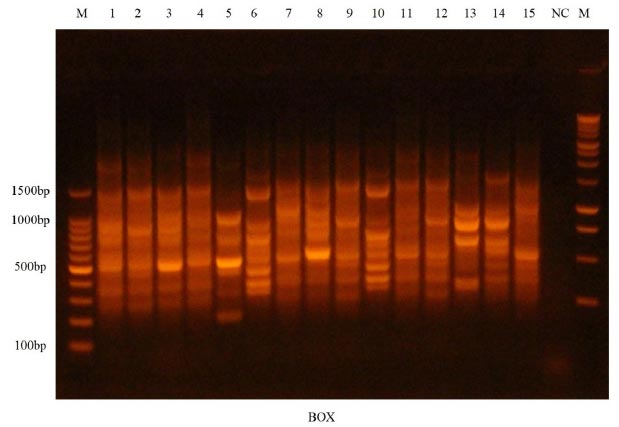
Gel electrophoresis for BOX-PCR profile of 15 pathogenic K. pneumoniae isolates using at 1.5% concentration. Lane M demonstrates 1.5 kb DNA ladders and lane NC demonstrates negative control.
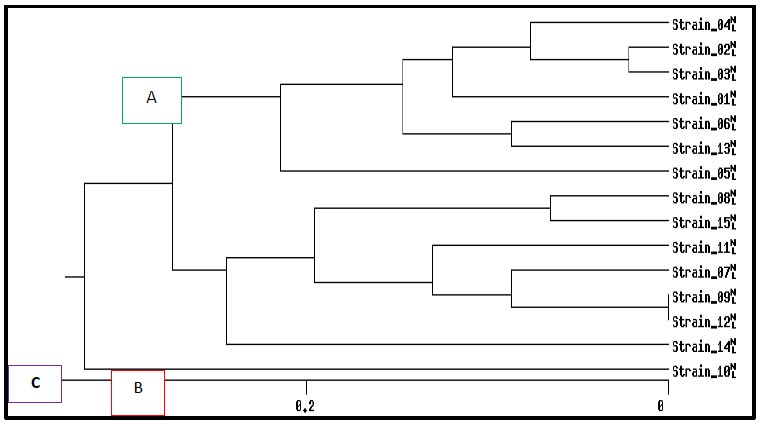
Dendrogram analysis for BOX-PCR products of fifteen pathogenic K. pneumoniae isolates based on UPGM method.
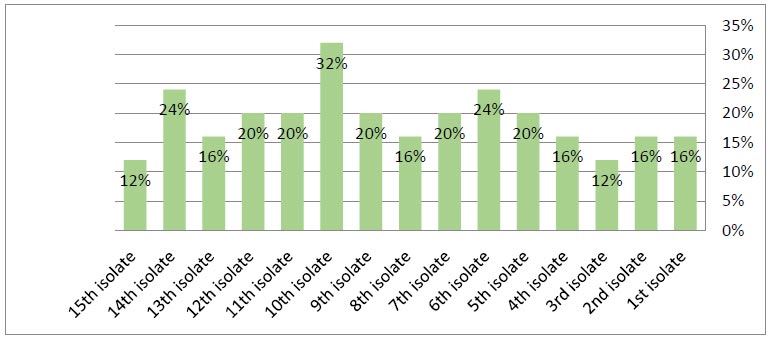
Frequency of BOXA-1R among MDR-Klebsiella pneumoniae isolates based on BOX-PCR technique.
in the isolates and their absence in the other isolates. As shown in Fig. (7), BOX-PCR typing showed three groups of genotypes and 15 unique isolates. Despite the differences in the isolation sources and location of these isolates, a strong clonality was observed, which indicated the epidemiology of these isolates. The results showed a genetic affinity between antibiotics-resistant K. pneumoniae isolated groups from various sources from Ramadi hospitals in Anbar province, while 1, 5, and 10 isolates had different genotypes, as illustrated (Fig. 8). Also, Fig. (8) illustrates the genetic repeats that indicate the diversity, similarity, and differences among K. pneumoniae isolates based on electrophoresis. These differences were caused by the genetic variety of K. pneumoniae isolates. On the other hand, 9 and 12 isolates were similar. The current study is in agreement with other studies [14, 59, 60] that K. pneumoniae is highly heterogeneous due to nucleotide sequence differences. BOX-PCR fingerprints also have fewer bands than BOXA1R since they use PCR rather than amplification. For BOX-PCR, the low number of bands can be a benefit due to the assay's ability to distinguish between minor genomic changes. Antibiotic resistance profiles are correlated with genotyping approaches; this is confirmed by many studies that are in line with Wasfi et al. [14], Ashayeri-Panah et al. [61], and Espinar et al. [62] regarding K. pneumoniae
CONCLUSION
Our study revealed a significant occurrence of K. pneumoniae strains with multidrug resistance (MDR), demonstrating resistance to multiple antimicrobial agents. Based on this current finding, K. pneumoniae clinical isolates exhibited a significant degree of antibiotic resistance heterogeneity. PCR genomic fingerprinting of the isolates using BOX repetitive sequences revealed a high degree of genetic variability. As a result, these isolates can circulate concurrently. Genetic variants in K. pneumoniae are a significant impediment to limiting the public health risk related to this pathogen. Hence, this diversity should be considered when developing measures for containing K. pneumoniae outbreaks. However, additional investigations are required to explore various epidemiological aspects of K. pneumoniae strains in our nation.
AUTHORS’ CONTRIBUTIONS
It is hereby acknowledged that all authors have accepted responsibility for the manuscript's content and consented to its submission. They have meticulously reviewed all results and unanimously approved the final version of the manuscript.
LIST OF ABBREVIATIONS
UTIs | = Urinary Tract Infections |
MDR | = Multidrug-resistant |
ERIC-PCR | = Enterobacterial Repetitive Intergenic Consensus Polymerase Chain Reaction |
ESBLs | = Extended-spectrum-β-lactamase |
ETHICS APPROVAL AND CONSENT TO PARTICIPATE
This study was approved by Ethical committee of University of Anbar (Ref. 9723), Iraq.
HUMAN AND ANIMAL RIGHTS
All human research procedures followed were in accordance with the ethical standards of the committee responsible for human experimentation (institutional and national), and with the Helsinki Declaration of 1975, as revised in 2013.